Simultaneous Nitrogen-Fixation and Photosynthesis in Trichodesmium
Introduction

By Katie Goulder
Trichodesmium is a marine cyanobacteria found near the surface of oligotrophic tropical and subtropical oceans and seas (Capone et al., 1997). It is known as “sea saw dust” since its colonies can be seen with the naked eye and have been mistaken for sandbars by ships, as first described by Captain Cook over 200 years ago (Figure 1). Trichodesmium can be found in various colors but the most common color is a yellow-brown due to the light absorbing pigment phycoerythrin, (Trichodesmium, [5]). However, T. erythraeum found in the Red Sea, takes on a red color when the blooms die out, and thus is an accepted origin of the name, the Red Sea (Colors from Bacteria, [6]).
Trichodesmium is a diazotrophic cyanobacteria, meaning it fixes nitrogen and performs photosynthesis. While there are other organisms that perform both processes, Trichodesmium is unique in that it evolves oxygen and fixes nitrogen simultaneously during the daylight hours without isolated intracellular structures or specific regions of nitrogen fixation within the colonies. This is remarkable as the O2 product of photosynthesis causes oxidative damage to the primary nitrogen fixing protein, nitrogenase, that deactivates the enzyme, and yet Trichodesmium is able to perform both processes without a problem (Capone et al., 1997). Because this bacteria can perform both photosynthesis and nitrogen fixation, it is involved in the carbon cycle in two ways: it makes direct contributions to carbon cycling as a primary producer and indirectly by fixing atmospheric nitrogen to stimulate growth of surrounding phytoplankton species (Orchard, et al., 2009). Trichodesmium is a model organism for diazotrophic cyanobacteria and has eight subspecies that have been used in research. However, for the purposes of this page, we will consider the general taxa of Trichodesmium.
Macroorganization
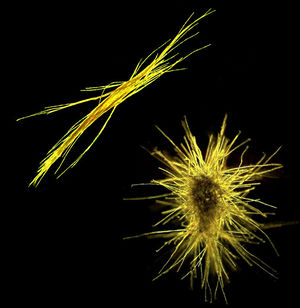
Trichodesmium grows in filaments of about 100-200 cells, referred to as a trichome, or trichoma. As individual cells divide, the trichome increases in length until cells in the middle of the trichome have a decrease in cellular metabolic activity as well as reduced C and N fixation. The trichome eventually splits to form 2 shorter trichoma that then continue the cycle. Groups of 100-200 trichoma then group together to form colonies (Finzi-Hart et al., 2009) that are in either a tuft-shaped (parallel) or puff-shaped (spherical) form (Figure 2). Both forms of colonies have a tightly compacted colonial core (Sheridan et al., 2002). Under conditions of low wind and high light, giant blooms can form that are 10-1000 km wide that are visible from space (Figure 1) (Trichodesmium, www.microbewiki.kenyon.edu).
Endogenous rhythms
In addition to its life cycle of breaking long chains, Trichodesmium maintains other cyclic characteristics such as its circadian rhythm of buoyancy and nutrient fixation. Buoyancy of Trichodesmium is dynamic and functions on a circadian cycle: Cells rise to remain closer to the ocean’s surface during the day and then sink during the night. This could be due to a ballasting effect from the accumulation of relatively dense carbohydrates during day since N2 and CO2 fixation only occurs when light is available. As these molecules accumulate, the cell becomes denser and sinks in the water column. Then at night, these products are consumed for processes such as biosynthesis and the cell becomes less dense and returns to the surface (Capone et al., 1997). Then the cycle continues during the day as metabolic products begin to build up again. The synergistic effects of the two endogenous cycles allows cells to fix CO2 and N2 on the surface, closer to the energy source, and then consume the products when photon energy cannot be collected.
Photosynthesis
While Trichodesmium are known for their unique ability to fix nitrogen, they are first and foremost photosynthetic bacteria. As such, they have evolved to utilize their high-energy environment using various pigments. Trichodesmium have pigments in photosystem II (PSII) called phycobilins that greatly outnumber chlorophyll. This ratio of phycobilins to chlorophyll is highly variable and is dependent on the presence of light as well as other environmental factors. Trichodesmium is rare in that it has 3 absorption peaks: Phycoerythrobilin (PEB) has absorption peaks at 545 and 565 nm fluorescence and phycourobilin (PUB) has an absorption peak at 495 nm. The ratio of PUB to PEB is also variable and increases at midday, when light conditions are high. During high light conditions, energy absorbed from PEB is transferred to PSII for O2 evolution. During low light conditions, PUB transfers energy to PEB and then on to PSII. The biophysical valve of excitation energy to PSII is thus dependent on light conditions.
In addition to varying concentrations of different pigments, Trichodesmium is also dynamic in its photosystem ratio. The 24:1 PSI to PSII ratio indicates that energy is generated in greater amounts through the cyclic electron transfer energy in PSI to provide the cell with ATP for nitrogen fixation without O2 evolution that occurs in PSII. This allows necessary energy to be produced without the harmful effects of oxygen production on nitrogen fixation.
This energetic valve has evolved to allow simultaneous fixation of N2 and O2 evolution. In addition to the skewed ratio of photosystems, Trichodesmium has evolved several other mechanisms by which they can down regulate the energy supply to PSII to prevent oxidative damage to the photosystems. The interconversion of PEB to PUB and the reverse reaction allows energy to be channeled to one photosystem or the other based on dynamic pigment ratios. The cells are also able to absorb light in carotenoids without transferring energy to PSII. On the community level, the colony organizational structure of Trichodesmium causes self-shading that limits light absorption. The gas vacuoles that provide controlled buoyancy also refract light and limit light absorption to cells closer to the surface. This effect is more prevalent in tufted colonies yet is still a factor of light absorption in both colony structures. By controlling the amount of light absorption and the flow of energy, Trichodesmium is able to allocate a portion of its energy resources to nitrogen fixation (Subramaniam et al., 1999).
Nitrogen Fixation
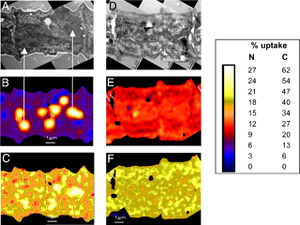
While Trichodesmium is not the only marine nitrogen-fixing cyanobacteria, it is unique in that nitrogen fixation and photosynthesis take place inside each cell simultaneously. Oxidation of nitrogenase destroys the function of the enzyme so that it can no longer fix nitrogen. Thus, simultaneous photosynthesis and nitrogen fixation is theoretically problematic. Other diazotrophic cyanobacteria employ different strategies to segregate the two processes. Gloeothece spp. performs photosynthesis during the day and fixes nitrogen at night to segregate the two processes temporally. Anabaena spp. has differentiated cells so that every tenth cell in its long chains is a heterocyst that has reduced photosynthetic mechanisms and instead fixes nitrogen that then diffuses to the surrounding photosynthetic cells (Finzi-Hart et al., 2009). However, Trichodesmium does not segregate the two processes as both processes occur in the same cell during the day when light is available. But how is this possible?
Multiple hypotheses have been proposed to explain this extraordinary phenomenon. These include unique nitrogenases that are resistant to O2 inactivation or the continuous production of nitrogenases to replace nitrogenases deactivated by O2 as soon as their function is destroyed. Other hypotheses include O2 consumption within the cell to allow intracellular O2 levels to be maintained at levels that allow nitrogen fixation or spatial segregation; either within each cell through trichome differentiation or between different regions of the colony. To date there is no evidence of diazocyte regions as different regions of the trichome do not differ in levels of nitrogen fixation and Trichodesmium nitrogenase protein turnover rates have been found to be slow (Capone et al., 1997).
However, there is recent evidence that has shed light on just how Trichodesmium are able to do it all. The cyanobacteria employ some temporal decoupling of N2 and CO2 fixation during the daylight hours. Photosynthesis has been found to occur at earlier hours of the day, with peak carbon fixation at about 10:00 am whereas nitrogen fixation occurs later in the day with a peak at about 12:00 am (Finzi-Hart et al., 2009). Because of this, we know that nitrogen fixation is oxygen dependent: low O2 partial pressure stimulates nitrogenase activity while high levels of O2 inhibit nitrogen fixation through nitrogenase oxidation.
There has also been evidence of intracellular spatial separation of the two metabolic processes. Finzi-Hart et al. incubated Trichodesmium cells with H13CO3 and 15N2 and then quantitatively imaged cells to determine 13C and 15N uptake using high-resolution secondary ion mass spectrometry (NanoSIMS). The authors noticed that the fixed 13C and 15N were stored in cyanophycin granules, which localizes the products during the day as seen in Figure 3 B and C respectively, only 8 hours after fixation. However, the localization is not a permanent state. The cyanophycin granules are in a dynamic state that allows the sequestered molecules to diffuse throughout the cell at night when nitrogen fixation has ceased for the day. This is depicted in Figure 3 in panels E and F for nitrogen and carbon respectively, as 24 hours after fixation the 13C and 15N are found in relatively equal concentrations throughout the cell. This supports the idea that fixation and localization of products occurs during the day and then the fixed products are used at night for biosynthesis. This experiment also provides evidence against the spatially differentiated cell hypothesis as the cyanophycin granules comprise about 6.4% of the cell volume and are evenly distributed throughout the trichoma. Since all cells have equivalent amount of storage space available, researchers believe that each cell performs the same amount of nitrogen fixation. The only cells that displayed a reduced amount of carbon and nitrogen fixation was in cells in the middle of the trichome that are likely beginning the process of programmed cell death (PCD) that leads to trichome splitting and is a regular part of the Trichodesmium life cycle (Finzi-Hart et al., 2009).
Micronutrients
In the oligotrophic zone, nutrients are scarce and can act as a limiting resource for microbial growth and metabolism. Iron, Fe, was originally thought to have been associated with nitrogenase function and thus could be a limiting factor for nitrogen fixation in Trichodesmium. However, N2 fixation rates and Trichodesmium biomass have been found to be independent of Fe concentration. This could be due to the fact that Fe is readily available in tropical and subtropical environments as it dissolves into the oceans from the atmosphere and thus is not a limiting factor (Sañudo-Wilhelmy et al., 2001).
However, phosphorus, P, has been found to be a limiting factor of nitrogenase function and ultimately of nitrogen fixation in Trichodesmium. While dissolved inorganic phosphorus (DIP) is the preferred source of phosphorus for marine bacteria due to its bioavailable form, dissolved organic phosphorus (DOP) can also be used by many microbes. DOP that contains phosphate esters comprises 75% of the DOP that is bioavailable in the water column of the diazotroph’s natural habitat. The ability to hydrolyze these bonds would allow Trichodesmium to inhabit a very specific niche in their environment. Trichodesmium utilize different transport systems during different periods of P availability: P-specific transport (Pst) is used in limited P conditions and the P-inorganic transport (Pit) system is used in P replete conditions. It has been found that regulatory enzymes phoX and phoA gene expression responded quickly (within 4 hours) to changing P conditions. Both enzymes are alkaline phosphatases and have different substrate specificity that allows for a greater diversity of DOP compounds to be hydrolyzed. The increased range of useful DOP for Trichodesmium allows the bacteria to extract P from the nutrient-poor environment that cannot be used by other organisms (Orchard et al., 2009). This lack of competition for a P source has allowed Trichodesmium to survive in high-energy yet nutrient poor conditions.
Ecology and Adaptations
Trichodesmium is found in oligotrophic tropical and subtropical oceans and seas that provide a very stable water column. These waters are characterized by low nutrient levels, clear water and deeply penetrating light. Trichodesmium have successfully adapted to survive in this environment through nitrogen fixation, as nitrogen frequently acts as a limiting resource for microorganism cell growth. The gas vacuoles present in Trichodesmium cells allow the bacteria to maintain natural buoyancy so that the cells can remain near the water surface to absorb more light photons. This counteracts the effects of wind, which can mix the top layer of the euphotic zone and cause the cells to be plunged deeper into the water column where light is not as abundant.
Trichodesmium has also evolved to have a photosynthetic apparatus that can tolerate very high levels of light to maximize the photon energy captured. This allows the cell to power nitrogen fixation, which has a very high energy demand. This apparatus also has photo protection, so that the high levels of light can be utilized instead of causing damage within the cell. The combined effect of the high energy and low nutrient conditions could also have acted as a selection pressure for the adaptation of a slow growth rate. Trichodesmium doubling time is any where from 3 to 5 days, which is slow in comparison to other cyanobacteria.
While Trichodesmium is affected by its environment, it also has effects on its surroundings. Trichodesmium blooms distort light penetration as well as the transfer of heat and gas between the surface of the ocean and the atmosphere. Nutrients, both organic and inorganic, accumulate in colonial blooms that can then act as a microhabitat for other organisms (see Symbiotic Relationships section). The fixed carbon and nitrogen enters the food web through leakage of organic nitrogen and other pathways but very little enters the food web by direct consumption. Some species of Trichodesmium produce a toxin that deters most grazers. However, some larger organisms have evolved resistance to this toxin and thus Trichodesmium has been found in the stomachs of some species of fish. A few studies have directly measured the amount of nitrogen fixed in Trichodesmium blooms and it is about three times as much as what occurs in the rest of the water column (Capone et al., 1997). Because the oligotrophic tropical and subtropical oceans and seas are so nutrient deficient, Trichodesmium is a vital component of this ecosystem and acts as the basis of microenvironments to support other microbial communities.
Symbiotic Relationships
Trichodesmium colonies are a stable physical structure for many other marine microorganisms to grow on. Specifically, 85% of puff and tuft structured colonies act as a floating island home for a varied mix of microorganisms. Trichodesmium colonies had more planktonic diversity and 2-6 orders of magnitude greater enrichment than microorganisms in the surrounding water column. The species diversity of the Trichodesmium colonies depended on various factors including mucoid matrix, colony integrity and colony morphology. Puff colonies were more likely to contain microorganisms and also had greater species richness than tuft colonies. Because Trichodesmium acts as structural foundation for bacterial growth, bacteria density increases rapidly and causes mucus membrane build up which in turn supports communities that include dinoflagellates, amoebae, diatoms and hydroids. This species richness increases both with mucous matrix thickness and colony stability. The mucous membrane of colonies also offers protection for some species including dinoflagellates, as well as increased prey concentrations for predator species such as hydroids (Sheridan et al., 2002). The structure of the Trichodesmium colonies acts as a micro ecosystem for microbial communities through its structure and form.
While Trichodesmium fixes N2 and evolves O2 for its own metabolism, it provides surrounding organisms with necessary and otherwise scarce nutrients necessary for growth to create a symbiotic community in the middle of the ocean. Trichodesmium leaks 30-50% of the nitrogen it has fixed into the water to provide biologically available nitrogen to other phytoplankton and other organisms living in the nitrogen poor conditions. It is estimated that Trichodesmium contributes about 5.7 Tmol of bioavailable nitrogen to the North Atlantic Ocean. This is equivalent to the amount of NO3- that originates from the ocean depths that is then mixed into the upper layers of the oligotrophic upper ocean (Finzi-Hart et al., 2009). In this way, Trichodesmium acts as a stable food source for a community of other microbes.
Impacts of Climate Change

Climate change is a hot topic in ecology right now and marine environments are not immune to its effects. The partial pressure of CO2 (pCO2) in the atmosphere is at its highest level in over half a million years (39 Pa) and if emissions are maintained at the current rate, pCO2 will continue to almost double to about 76 Pa by 2100. While this has obvious direct impacts on carbon cycling, it also has indirect effects on nitrogen cycling that could be just as important. Nitrogen fixation affects the carbon cycle because as the amount of fixed nitrogen increases, other photosynthetic organisms are provided the necessary nutrients to grow, causing an increase in CO2 sequestration so that some of the CO2 emissions can be sequestered that can help alleviate the growing Green House effect. This was confirmed in Hutchins et al., who found that high atmospheric CO2 levels increased N2 as well as CO2 fixation (Figure 4 A and B). The authors also found that CO2 acts as an umbrella limiting factor of nitrogen fixation that can mask the effects of low micronutrient content, such as phosphorus. The increase in nitrogen fixation at higher CO2 levels may be an indirect effect of the lack of CO2 stress. Also, while the effects of P limitations were not as severe in environments with increased levels of CO2, CO2 levels did not effect the incorporation of phosphorus in regular Trichodesmium metabolism.
The increase in atmospheric CO2 causes increased Green House Effect that is in turn causing global temperatures to rise. While global warming is highly controversial, the increase in temperature had no effect on nitrogen fixation rates. However, the increase in temperature is projected to expand the area of suitable habitat for Trichodesmium by 27%. In this way, temperature will have more of an indirect affect on nitrogen fixation in that there will be greater biomass of Trichodesmium so that nitrogen is being fixed at the same rate but there is more Trichodesmium to do the fixing. The rise in temperature also caused a small increase in CO2 fixation. The combined effect of higher CO2 levels and increase temperature gave evidence to increased efficiency of harvesting photon energy to power both O2 evolution and N2 fixation (Hutchins et al., 2007).
While this study cannot be used to predict the future, it gives hope that as humans continue to take advantage of the earth’s resources and cause further damage to our planet through excessive CO2 emissions, Trichodesmium have the ability to increase their fixation rates and to help counteract the pollution. The multitude of microbes employed to help clean up human pollution of all kinds is quite impressive and it demonstrates how incredibly diverse microbe metabolisms are. However, the question remains, what is the threshold of anthropogenic activity that can’t be saved by our invisible community?
References
[7] [Capone, D.G., Zehr, J.P., Paerl, H.W., Bergman, B. & Carpenter, E.J. Trichodesmium, a globally significant marine cyanobacterium. Science. 1997. Volume 276. p. 1221- 1229.]
[8] [Finzi-Hart, J.A., Pett-Ridge, J., Weber, P.K., Popa, R., Fallon, S.J., Gunderson, T., Hutcheon, I.D., Nealson, K.H. & Capone, D.G. Fixation and fate of C and N in the cyanobacterium Trichodesmium using nanometer-scale secondary ion mass spectrometry. Proceedings of the National Academy of Sciences of the United States of America. 2009. Volume 106. Issue 15. p. 6345-6350.]
[9] [Hutchins, D.A., Fu., F.X., Zhang, Y., Warner, M.E., Feng, Y., Portune, K., Bernhardt, P.W., & Mulholland, M.R. CO2 control of Trichodesmium N2 fixation, photosynthesis, growth rates, and elemental ratios: implications for past, present, and future ocean biogeochemistry. Limnology and Oceanography. 2007. Volume 52. Issue 4. p. 1293-1304.]
[10] [Orchard, E. O., Webb, E. A. & Dyhrman, S.T. Molecular analysis of the phosphorus starvation response in Trichodesmium spp. Environmental Microbiology. 2009. Volume 11. Issue 9. p. 2400-2411.]
[11] [Sañudo-Wilhelmy, S.A., Kustka, A.B., Gobler, C.J., Hutchins, D.A., Yang, M., Lwiza, K., Burns, J., Capone, D.G., Raven, J.A. & Carpenter, E.J. Phosphorus limitation of nitrogen fixation by Trichodesmium in the central Atlantic Ocean. Nature. 2001. Volume 411. p. 66-69.]
[12] [Sheridan, C.C., Steinburg, D.K. & Kling, G.W. The microbial and metazoan community associated with colonies of Trichodesmium spp.: a quantitative survey. Journal of Plankton Research. 2002. Volume 24. Issue 9. p. 913-922.]
[13] [Subramaniam, A., Carpenter, E.J., Karentz, D., Falkowski, P.G. Bio-optical properties of the marine diazotrophic cyanobacteria Trichodesmium spp. Absorption and photosynthetic action spectra. Limnology and Oceanography. Volume 44. Issue 3. p. 608-617.]
[14] "Microbe Wiki" Trichodesmium
[15] "Causes of Color" Colors From Bacteria
Edited by student of Joan Slonczewski for BIOL 238 Microbiology, 2015, Kenyon College.