The Role of the Gut Microbiome in the Development of Neurological Diseases
Introduction
The gut microbiome is one of the most important collections of microbiota in the human body. The trillions of bacteria, archaea, and fungi that comprise and inhabit the intestinal tract help to manage and direct vital aspects of immunity, development, and metabolism within the body. The human microbiome began to be studied by the likes of Theodor Escherich, Henry Tissier, and Alfred Nissle in the late nineteenth century and early twentieth century. However, the scale of the gut microbiome–and the microbiome as a whole–was not truly known until the Human Microbiome Project Consortium published their sequencing of thousands of human gut microbiomes in 2012 [1] . It has been estimated that the density of bacterial cells within the colon is somewhere between 1011 and 1012 per milliliter [2]. Now, researchers are just beginning to understand how our microbiota influences the rest of our body, and how the collection of microbes can contribute to the development of neurological diseases.
Composition and Genetics of the Gut Mircrobiome
Each person contains a unique and personal microbial community that is acquired from birth, developing rapidly until the age of three and then slowly changing over the rest of an individual’s life [3]. These slow changes can be caused by lifestyle choices, such as diet, medication, exercise, as well as other factors. The microbiome of an individual is acquired immediately from birth and develops quickly and intensely throughout infancy. As an infant has direct contact with their mother through birth and breastfeeding, much of the microbiome is inherited from the mother. In a mature microbiome, the most abundant gut microbial phyla are Firmicutes, Bacteroidetes, Actinobacteria, Proteobacteria, Fusobacteria, and Verrucomicrobia, with Firmicutes and Bacteriodetes making up approximately 90% of the bacterial population within the gut [4].
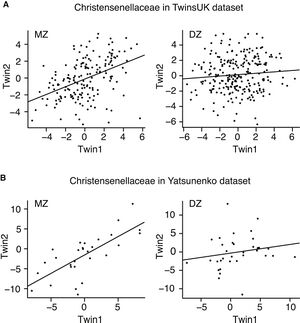
Although the microbial community is not inherited through Mendelian patterns, there is current research suggesting that there may be ways our genetic code directly influences the gut microbiome. In a study conducted by researchers in the UK, it was found that monozygotic (identical) twins have similar microbiomes as compared to dizygotic (fraternal) twins [5]. The most heritable taxa was found to be Christensenellaceae, with monozygotic twins showing greater similarity in levels of the bacterial family as compared to dizygotic twins [5]. However, little is known about the biological mechanisms that causes this, prompting need for further research. It is known that higher levels of Christensenellaceae are associated with a lower BMI, displaying another way that obesity factors can be inherited [6]. Once the alleles that determine relative levels of Christensenellaceae are found and studied, the ability for the use and exploitation of Christensenellaceae to potentially treat obesity and other health issues will be available.
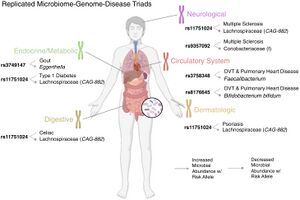
Microbial-associated variants (MAVs) are another (and more studied) instance in which genetics can directly affect the microbial community, thereby affecting the health of the individual. MAVs are caused by single nucleotide polymorphisms that then cause the relative abundance of certain taxa within the gut to change, for reasons unknown [7]. These single nucleotide polymorphisms are then passed down through generations in the form of Mendelian alleles. Certain MAVs have been linked to endocrine, digestive, neurological, cardiovascular, and dermatological conditions [7]. Some of these conditions include multiple sclerosis, celiac disease, type 1 diabetes, and pulmonary heart disease.
Development of Neurological Diseases
Gut-brain axis
The gut microbiome plays an important role in what is known as the gut-brain axis (GBA). The GBA is a bidirectional communication system between the central and enteric (intestinal) nervous systems [8]. The GBA links the cognitive and emotional hubs of the brain to the intestinal functions. More recent research has discovered that gut microbiota influence the GBA through their own signaling pathways [8]. The autonomic nervous system, hypothalamic-pituitary-adrenal (HPA) axis, and the nerves within the intestinal tract are all subject to influence by both signals from the brain and from the microbes living within the gut [9].
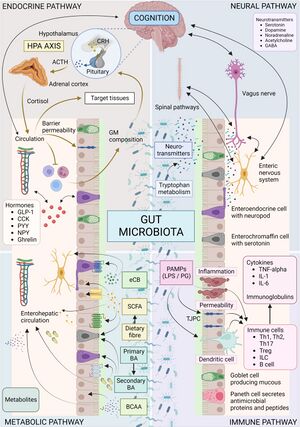
One of the most influential pathways between gut microbiota and the brain is through the HPA axis. The HPA axis is responsible for the release and regulation of cortisol within the body through corticotropin-releasing factor from the hypothalamus and adrenocorticotropic hormone secretion from the pituitary gland, which then causes the adrenal glands to release cortisol [8]. Cortisol acts upon gastrointestinal cells and can cause dysbiosis of the gut microbiome [10]. This dysbiosis can lead to a decrease of short chain fatty acids, which are produced by gut microbiota in the processing of non-digestible polysaccharides [10]. It has also been shown that short chain fatty acids help to downregulate the HPA axis by reducing the cortisol response within the body [10]. High cortisol levels can lead to imbalances within the gut microbiome, which in turn can cause higher cortisol secretion levels through the decrease of short chain fatty acids produced by the gut microbiota.
Parkinson’s
The gut microbiome has been linked to the development of Parkinson’s in individuals. Parkinson’s–a neurodegenerative disease that is thought to be caused by both environmental and genetic factors–leads to the loss of dopaminergic neurons, eventually leading to brain atrophy [11]. One of the pathways that causes Parkinson’s is the pathogenic mutation of alpha-synuclein protein into amyloid fibrillar structures, which activates neurodegeneration [11]. It has been found that alpha-synuclein protein aggregates are found in both the substantia nigra and the enteric nervous system [11], suggesting that the pathological pathway that leads to Parkinson’s disease may begin in the gut. It has been shown that patients with Parkinson’s disease have district gut microbiome compositions when compared to those without the disease [12]. Specifically, there were altered abundances of the Bifidobacteriaceae, Christensenellaceae, Lachnospiraceae, Lactobacillaceae, Pasteurellaceae, and Verrucomicrobiaceae families in patients with Parkinson’s disease [12].
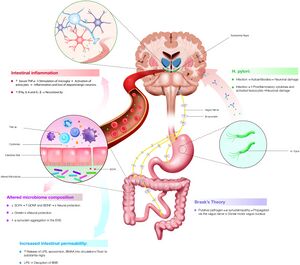
In the early 2000s, German anatomist Heiko Braak published his hypothesis, which suggests a pathogenetic mechanism in which sporadic Parkinson’s develops. In his hypothesis, an unknown pathogen enters the body and ends up in the gastric epithelial lining [14]. Within the gut, the pathogen causes gut neurons to produce an increase in the formation of misfolded alpha-synuclein protein [14]. These misfolded proteins are then transported to the nervous system where they take root in the brain, causing the loss of dopaminergic neurons [14]. More recent research builds upon Brakk’s hypothesis by proposing that gut dysbiosis plays a role in why gut neurons produce the misfolded alpha-synuclein protein. The proposed pathogen causes dysbiosis of the gut microbiota, leading to an increase in the number of bacterial amyloids [15]. These amyloids then interact with the gut neurons, leading to the alpha-synuclein protein being misfolded [15]. It has been shown that there are many bacterial species that make up the human microbiome which are capable of assembling amyloids, including members of the Streptococcus, Staphylococcus, Salmonella, Mycobacteria, Klebsiella, Citrobacter, and Bacillus families [15]. It has been hypothesized that perhaps one of the species belonging to this family holds the key to understanding the linkage between the gut microbiome and the development of sporadic Parkinson’s disease.
There also may be evidence suggesting that non-sporadic Parkinson’s disease is in part caused by the gut microbiome. One of the comorbidities with Parkinson’s disease is rapid eye movement sleep behavior disorder (RBD), with more than about half of Parkinson’s disease patients also having RBD [16]. RBD is typically seen in humans earlier than Parkinson’s, being a great warning sign that Parkinson’s might develop in the individual. In a study conducted in 2020, the gut microbiome of RBD patients with no signs of neurodegenerative disease was found to have significant overlap in the microbial signature with the gut microbiome of Parkinson’s patients, with a prominent abundance of Verrucomicrobiaceae that is not seen in the gut microbiome of healthy individuals [13]. A better understanding of the relationship between the microbial signatures of RBD patients and patients with Parkinson’s could lead to preventative measures being taken by RBD patients to prevent the onset of Parkinson’s [13]. For these RBD patients, it has been suggested that one way to take preventative measures against Parkinson’s is through the use of probiotics that promote a healthy microbial signature within the gut [13].
Alzheimer’s
The gut microbiome has also been linked to both the development and possible treatment of Alzheimer’s. Alzheimer’s is characterized by brain atrophy caused by the accumulation of amyloid beta in the neurons and the hyper-phosphorylation of the tau protein in the dendrites and axons of cortical neurons [17]. Recent research suggests that chronic inflammation in the brain can lead to the development of Alzheimer’s [18]. This chronic inflammation can be caused by cytokines released by the central nervous system, or the increased activity of microglia in the brain [18]. To mitigate this, some diets such as the Mediterranean diet and dietary approaches to stop hypertension (DASH) have been studied retrospectively in populations, with lower rates of Alzheimer’s disease being seen in both [19]. The mechanism for why there were lower rates is strongly hypothesized to be an increase in short-chain fatty acids produced by gut bacteria [20]. Both the Mediterranean and DASH diets are high in fiber, which is able to be converted into short-chain fatty acids, which have anti-inflammatory properties [20].
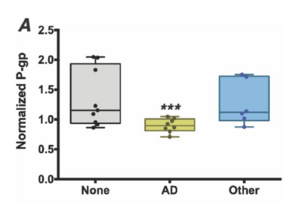
The microbial signature of the gut microbiome has also been hypothesized to potentially lead to Alzheimer’s disease. The P-glycoprotein (P-gp) is known to be involved in protection of both the epithelial cells of the gut and the blood-brain barrier [22]. In patients with Alzheimer’s, it has been shown that members of the taxons Bacteroides, Alistipes, Odoribacter, and Barnesiella are more common while members of the taxon Lachnoclostridium are less common [23]. Furthermore, supernatents from stool samples that came from Alzehimer’s patients induced a significantly lower expression of functional P-gp than did from the supernatents of patients with no dementia or other dementia types [24]. Dysfunctional P-gp is linked to higher rates of amyloid beta accumulation in the brain [24]. These data points suggest that the difference in microbial abundance could be linked to the levels of functional P-gp, which in turn corresponds to the development of Alzheimer’s. It has also been seen that bacteria populating the gut microbiome can excrete large quantities of amyloids and lipopolysaccharides (LPSs) [21]. These molecules may lead to the development of Alzheimer’s during the process of aging–when the gastrointestinal tract epithelium and the blood-brain barrier become increasingly permeable–through the release of cytokines caused by LPS/amyloid presence [21]. Even further, the lipopolysaccharide produced by Bacteroides fragilis (a common gut microbe) is an extremely potent inducer of the pro-inflammatory transcription factor NFκB (p50/p65) complex when exposed to human brain cells [25]. That transcription factor is a known initiator involved in inflammatory neurodegeneration in the development of Alzheimer’s disease [25].
Treatment of other neurological diseases
There have been numerous studies that support the use of probiotics or antibiotics in the treatment of neurological diseases. One of the most compelling studies that has been replicated and repeated deals with the use of antibiotics in the treatment of hepatic encephalopathy, a brain disorder that is a result of cirrhosis of the liver in which toxins are built up within the bloodstream, affecting the brain [26]. The use of antibiotics such as neomycin, metronidazole, and rifaximin help to reduce the number of toxin-producing bacteria within the gut and therefore help to treat hepatic encephalopathy [27].
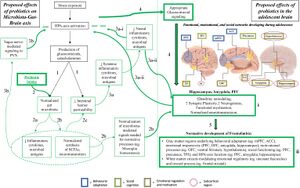
A more recent and novel study utilized probiotics containing the strain Lactobacillus Plantarum PS128 to treat depression in adolescents [28]. The bacteria helped to downregulate the HPA-axis through the production of healthy levels of short chain fatty acids. This downregulation helped to mitigate depressive symptoms in adolescents, as the HPA-axis is hyperactive in adolescent depression [28].
References
- ↑ The Human Microbiome Project Consortium. Structure, function and diversity of the healthy human microbiome. Nature. 2012 Jun;486(7402):207–14.
- ↑ Bessesen DH. Human gut microbes associated with obesity. Yearbook of Endocrinology. 2007 Jan;2007:163–5.
- ↑ Yatsunenko T, Rey FE, Manary MJ, Trehan I, Dominguez-Bello MG, Contreras M, et al. Human gut microbiome viewed across age and geography. Nature [Internet. 2012 May 9;486(7402):222–7. Available from: https://www.nature.com/articles/nature11053]
- ↑ Arumugam M, Raes J, Pelletier E, Le Paslier D, Yamada T, Mende DR, et al. Enterotypes of the human gut microbiome. Nature. 2011 Apr 20;473(7346):174–80.
- ↑ 5.0 5.1 5.2 Goodrich Julia K, Waters Jillian L, Poole Angela C, Sutter Jessica L, Koren O, Blekhman R, et al. Human Genetics Shape the Gut Microbiome. Cell. 2014 Nov 6;159(4):789–99.
- ↑ Mazier W, Le Corf K, Martinez C, Tudela H, Kissi D, Kropp C, et al. A New Strain of Christensenella minuta as a Potential Biotherapy for Obesity and Associated Metabolic Diseases. Cells [Internet. 2021 Apr 1 [cited 2022 Oct 29];10(4):823. Available from: https://www.mdpi.com/2073-4409/10/4/823/htm]
- ↑ 7.0 7.1 7.2 Markowitz RHG, LaBella AL, Shi M, Rokas A, Capra JA, Ferguson JF, et al. Microbiome-associated human genetic variants impact phenome-wide disease risk. Proceedings of the National Academy of Sciences. 2022 Jun 24;119(26).
- ↑ 8.0 8.1 8.2 Carabotti M, Scirocco A, Maselli MA, Severi C. The gut-brain axis: interactions between enteric microbiota, central and enteric nervous systems. Annals of Gastroenterology : Quarterly Publication of the Hellenic Society of Gastroenterology [Internet. 2015 Apr;28(2):203. Available from: https://pmc.ncbi.nlm.nih.gov/articles/PMC4367209/]
- ↑ Appleton J. The Gut-Brain Axis: Influence of Microbiota on Mood and Mental Health. Integrative Medicine: A Clinician’s Journal [Internet. 2018 Aug;17(4):28. Available from: https://pmc.ncbi.nlm.nih.gov/articles/PMC6469458/]
- ↑ 10.0 10.1 10.2 10.3 Rusch JA, Layden BT, Dugas LR. Signalling cognition: the gut microbiota and hypothalamic-pituitary-adrenal axis. Frontiers in Endocrinology [Internet. 2023 Jun 19;14. Available from: https://pmc.ncbi.nlm.nih.gov/articles/PMC10316519/]
- ↑ 11.0 11.1 11.2 Srinivasan E, Chandrasekhar G, Chandrasekar P, Anbarasu K, Vickram AS, Karunakaran R, et al. Alpha-Synuclein Aggregation in Parkinson’s Disease. Frontiers in Medicine [Internet. 2021 Oct 18;8:736978. Available from: https://www.ncbi.nlm.nih.gov/pmc/articles/PMC8558257/]
- ↑ 12.0 12.1 Hill-Burns EM, Debelius JW, Morton JT, Wissemann WT, Lewis MR, Wallen ZD, et al. Parkinson’s disease and Parkinson’s disease medications have distinct signatures of the gut microbiome. Movement Disorders. 2017 Feb 14;32(5):739–49.
- ↑ 13.0 13.1 13.2 13.3 Elfil M, Kamel S, Kandil M, Koo BB, Schaefer SM. Implications of the Gut Microbiome in Parkinson’s Disease. Movement Disorders. 2020 Feb 24;35(6).
- ↑ 14.0 14.1 14.2 Braak H, de Vos RAI, Bohl J, Del Tredici K. Gastric α-synuclein immunoreactive inclusions in Meissner’s and Auerbach’s plexuses in cases staged for Parkinson’s disease-related brain pathology. Neuroscience Letters. 2006 Mar;396(1):67–72.
- ↑ 15.0 15.1 15.2 Friedland RP, Chapman MR. The role of microbial amyloid in neurodegeneration. PLoS Pathogens [Internet. 2017 Dec 21 [cited 2021 May 19];13(12). Available from: https://www.ncbi.nlm.nih.gov/pmc/articles/PMC5739464/]
- ↑ Zhang X, Sun X, Wang J, Tang L, Xie A. Prevalence of rapid eye movement sleep behavior disorder (RBD) in Parkinson’s disease: a meta and meta-regression analysis. Neurological Sciences. 2016 Oct 21;38(1):163–70.
- ↑ National Institute on Aging. Alzheimer’s disease fact sheet [Internet. National Institute on Aging. National Institutes of Health; 2023. Available from: https://www.nia.nih.gov/health/alzheimers-and-dementia/alzheimers-disease-fact-sheet]
- ↑ 18.0 18.1 Newcombe EA, Camats-Perna J, Silva ML, Valmas N, Huat TJ, Medeiros R. Inflammation: the link between comorbidities, genetics, and Alzheimer’s disease. Journal of Neuroinflammation. 2018 Sep 24;15(1).
- ↑ McGrattan AM, McGuinness B, McKinley MC, Kee F, Passmore P, Woodside JV, et al. Diet and Inflammation in Cognitive Ageing and Alzheimer’s Disease. Current Nutrition Reports. 2019 Apr 4;8(2):53–65.
- ↑ 20.0 20.1 National Institute on Aging. Beyond the brain: The gut microbiome and Alzheimer’s disease [Internet. National Institute on Aging. 2023. Available from: https://www.nia.nih.gov/news/beyond-brain-gut-microbiome-and-alzheimers-disease]
- ↑ 21.0 21.1 21.2 Lin L, Zheng LJ, Zhang LJ. Neuroinflammation, Gut Microbiome, and Alzheimer’s Disease. Molecular Neurobiology. 2018 Mar 9;55(11):8243–50.
- ↑ Medicines interactions: the role of P-glycoprotein [Internet. www.medsafe.govt.nz. 2011. Available from: https://www.medsafe.govt.nz/profs/puarticles/p-glycoproteinsept2011.htm]
- ↑ Tiwari P, Dwivedi R, Bansal M, Tripathi M, Dada R. Role of Gut Microbiota in Neurological Disorders and Its Therapeutic Significance. Journal of Clinical Medicine. 2023 Feb 19;12(4):1650.
- ↑ 24.0 24.1 Haran JP, Bhattarai SK, Foley SE, Dutta P, Ward DV, Bucci V, et al. Alzheimer’s Disease Microbiome Is Associated with Dysregulation of the Anti-Inflammatory P-Glycoprotein Pathway. mBio [Internet. 2019 Jun 25 [cited 2020 Jun 30];10(3).]
- ↑ 25.0 25.1 Lukiw WJ. Bacteroides fragilis Lipopolysaccharide and Inflammatory Signaling in Alzheimer’s Disease. Frontiers in Microbiology. 2016 Sep 26;7.
- ↑ Mandiga P, Foris LA, Kassim G, Bollu PC. Hepatic encephalopathy [Internet. Nih.gov. StatPearls Publishing; 2019.]
- ↑ Patidar KR, Bajaj JS. Antibiotics for the Treatment of Hepatic Encephalopathy. Metabolic brain disease [Internet. 2013 Jun 1;28(2):307–12.]
- ↑ 28.0 28.1 28.2 28.Freimer D, Yang TT, Ho TC, Tymofiyeva O, Leung C. The gut microbiota, HPA axis, and brain in adolescent-onset depression: Probiotics as a novel treatment. Brain, Behavior, & Immunity - Health. 2022 Oct;26:100541.
Edited by Owen Gingras, student of Joan Slonczewski for BIOL 116, 2024, Kenyon College.