The Temperature Relationship of Batrachochytrium dendrobatidis
Introduction
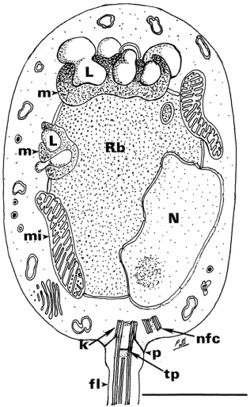
By [Eva Brazer]
Amphibian species around the world are experiencing unprecedented population decline due to the emerging infectious disease chytridiomycosis, which is caused by the chytrid fungus Batrachochytrium dendrobatidis (Bd) (Fig 1).[2] The chytrid pathogen is considered an emerging infectious disease because it was discovered and described only in the last twenty years,[3] and has continued to spread globally causing devastating effects.[4] Batrachochytrium dendrobatidis has been identified on every continent on which amphibians are present (Fig 4).[2]
Recent global mapping initiatives reported that Batrachochytrium dendrobatidis had been identified as having infected 1,015 of the 1,854 species examined (54%). Further population analysis found that the fungus has caused the decline of at least 501 species (6.5% of all amphibian species), with 124 experiencing a decrease in the species population of over 90%.[5] Over one hundred presumed extinctions have been attributed to chytridiomycosis.[6] The catastrophic impact of Batrachochytrium dendrobatidis is so significant that it is believed to be the largest threat to the biodiversity of any class of vertebrate.[2] Batrachochytrium dendrobatidis has been documented in hundreds of amphibian species, and reports of infection in new species and geographic locations are continually increasing.[6]
Climate change models are predicting an increase in the frequency and magnitude of temperature fluctuations.[7] This has caused concern that these temperature fluctuations may lead to increased pathogen development, transmission, and ectotherm-host susceptibility.[8] The parasitic performance of Batrachochytrium dendrobatidis is sensitive to temperature.[8] By examining the relationship between pathogen and host interactions, particularly in relation to temperature fluctuations, scientists can better predict and mitigate the spread of Batrachochytrium dendrobatidis to new amphibian populations.
Phylogeny
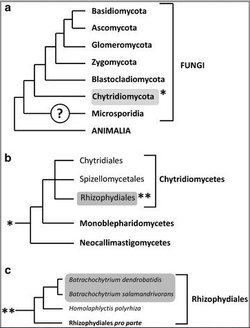
Scientific classification
Kingdom: Fungi
Division: Chytridiomycota
Class: Chytridiomycetes
Order: Rhizophydiales
Genus: Batrachochytrium
Species: B. dendrobatidis
After its discovery, the amphibian chytrid fungus was placed in a new genus, Batrachochytrium, which was originally in the order Chytridiales.[10] The designation of the fungus was initially determined by examining the zoospore ultrastructure (Fig 1).[1] However, the discovery of new ultrastructural and molecular characteristics helped to segregate different orders, designating Batrachochytrium dendrobatidis as a member of the order Rhizophydiales (Fig 2).[11] This separation was supported by analysis of the small subunit ribosomal DNA (ssu-rDNA) sequence of the pathogen. At the time of its designation, Batrachochytrium dendrobatidis was the only known member of its genus.[10] It was also the only known member of Chytridiomycota to parasitize vertebrates,[6] and is one of a very limited number of endobiotic fungi in the order Rhizophydiales.[12]
It was not until 2013 that another member of the Batrachochytrium genus became known. A second pathogenic species, Batrachochytrium salamandrivorans (Bsal) was discovered.[5] Similarly to B. dendrobatidis, B. salamandrivorans causes chytridiomycosis and death in its host; however, in this case the species preferentially infects salamanders.[13]
Infection of Host
Chytridiomycosis is caused by an aquatic flagellated fungus that targets amphibian hosts.[6] Batrachochytrium dendrobatidis colonizes the superficial epidermis, resulting in a thickening of the outer layer of skin. While cutaneous fungal infections like chytridiomycosis are rarely fatal in vertebrate species, amphibians are particularly susceptible due to the unique physiological qualities of their skin.[1] In amphibians, the skin plays a crucial role in regulating the exchange of respiratory gases, water and electrolytes. By disrupting the epidermal layer, Batrachochytrium dendrobatidis can cause complications in osmoregulation, hydration, breathing, and thermoregulation.[6] In susceptible species, death usually occurs between 18 and 70 days after initial exposure. The incubation time varies with species, amount of exposure and temperature.[1] Relatively resistant amphibian species that show no clinical signs often still carry light infections and act as asymptomatic carriers, spreading the disease.
In tadpoles, keratin is localized to the mouthparts, but after metamorphosis the skin of the mature amphibian becomes keratinized. Because Batrachochytrium dendrobatidis targets keratinized areas of the organism, the detrimental impact of the fungal infection is more pronounced in the post-metamorphic stages, often resulting in death. In tadpoles the localization of infection to the mouthparts often results in sublethal effects. However, damage to the mouthparts can limit nutrient uptake and impair growth.[1][2]
In amphibians, clinical signs of chytridiomycosis at the cellular level include damaged nuclei, altered cytoplasm and most significantly, a hyperplastic response,[14] a very common response to epidermal injury or infection.[1] Hyperplasia causes excessive skin sloughing due to increased turnover of epidermal cells and swelling of the cells nearest the foci of infection.[14] This abnormal molting is significant because it can be an indication of the mortality risk for chytridiomycosis. If the species has thick skin and therefore a sufficient supply of epidermal layers, epidermal cell turnover can increase to rid the amphibian of all infected cells.[12] However, thinning of the epidermis may occur if epidermal cells are not regenerated at a rate consistent with the rapid rate at which cells are sloughing from the surface. Conversely, if the rate of regeneration exceeds the rate of cell turnover, buildup can occur, impeding cutaneous functions.[6] If the disruption of normal physiological processes in the epidermis is significant enough, the resulting osmotic imbalance and electrolyte depletion can be fatal.[12] The interference of the fungus with ion transport functions in the epidermis eventually results in cardiac arrest and death.[15] At the macroscopic level, signs like lethargy, anorexia, loss of reflexes and sometimes-abnormal posture is observed, although this is generally only observed immediately before death.[12]
Life Cycle
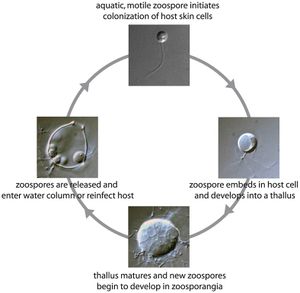
Batrachochytrium dendrobatidis is an aquatic fungus that requires a wet surface to reproduce. The fungus is associated with the colonization of the epidermis of adult amphibians and the mouthparts of tadpoles by a spherical eukaryotic pathogen.[7] The life cycle of Batrachochytrium dendrobatidis has two main stages,[1] the motile zoospore stage for dispersal and a stationary thallus stage that develops into a sporangium for asexual replication.[12]
The cycle begins with the motile zoospore, which travels using a flagellum through the water until it finds an amphibian host.[6] Zoospores are the waterborne, unwalled, and flagellated state of the fungus.[1] The spherical body (soma) of the zoospore is 3 – 5 μm in diameter with a posteriorly directed flagellum (19–20 μm in length).[10] The zoospore initiates colonization of the skin by burrowing into the epidermis cells and encysts (the spore stops moving, absorbs the flagellum, and forms a cell wall), developing into a spherical thallus.[12] A germination tube protrudes from the thallus wall and extends into the host's tissue down into the granular layer (stratum granulosum). The thallus produces an urn-shaped zoosporangium, inside of which new zoospores are produced through asexual reproduction.[6] The immature zoosporangia begin development in the deeper, more viable epidermal cells, where sporangia induces premature death and keratinization of the host cell. As a result the zoosporangia finishes development in a keratinized cell lacking organelles. Batrachochytrium dendrobatidis has adapted to live in the stratified epidermis and has a rate of development that coincides with the maturation of the cell.[1] During sporangial development, discharge tubes form, protruding out of the cell to the skin's surface. Once open to the surface, the discharge papilla opens, releasing the mature zoospores.[15] However, if Batrachochytrium dendrobatidis senses that the environment around the host is too dry for the zoospores to be released, the zoosporangia may retain them until there is adequate moisture to induce their discharge.[1] The new zoospores are released after a temperature-dependent generation time of 4 to 10 days,[7] restarting the cycle as they enter the water column or reinfect the host (Fig 3).[1]
Batrachochytrium dendrobatidis persists at a wide range of permissive and optimum temperatures allowing the pathogen to survive in many environments.[4] The optimal growth range for the fungus is between 17° C and 25° C, with a critical thermal maxima of 30° C, and a critical thermal minima of 4° C.[7] While the fungus can grow at a wide range of temperatures, environmental temperature can significantly alter the performance of the pathogen. After they are released, the zoospores remain active for only 24 hours at 23° C; however, at 4-14° C, they survive twice as long, remaining active for 48 hours.[4] At colder temperatures (i.e. <17° C), Batrachochytrium dendrobatidis zoosporangia develop and mature slower, however they produce more and longer-living zoospores.[7]. At higher temperatures (i.e. >25° C), an increased rate of turnover for epidermal cells and reduced growth and development of zoospores may result in ridding the host of the infection as the fungus living in the epidermis is shed before it has completed its life cycle.[1] Zoospores are the primary mode of dispersal for Batrachochytrium dendrobatidis, but they are constrained in the distance they can travel and the limited time before they encyst.[4] The fungus is often found in clusters on the skin,[1] this may occur because zoospores are attracted to the site of infection, or because zoospores are released and immediately encyst, infecting the adjacent area.[4] After they were released, the zoospores in culture swam, on average, less than 2 cm before encysting.[7] However, zoospores are capable of chemotaxis, which allows them to direct their movement through the water column towards a host through preferential attraction to keratin.[15] Without chemotaxis, it is unlikely the zoospores released in the water would come in contact with a host due to their low concentration.[4] While Batrachochytrium dendrobatidis colonizes keratinized epidermal cells, it is not known if it actually degrades and ingests the keratin for nutrients, or whether it is simply found in keratinized epidermal cells because they are the simplest to access and parasitize.[12][1]
Immunity
Unlike most fungal pathogens,[15] Batrachochytrium dendrobatidis is confined to the keratinized epidermis and tadpole mouthparts of its host and does not infect other organs. Because chytridiomycosis infects only the skin, having immune defenses in the skin are crucial for survival.[16] The first line of defense against infectious zoospores is secreted antimicrobial peptides in the skin mucus that inhibit growth. In addition to this innate defense by the host, the mucus also contains symbiotic skin bacteria.[15] Some of these symbiotic bacteria, like Janthinobacterium lividum,[17] produce antifungal metabolites. The final defense against chytridiomycosis infection is the lymphocyte-mediated adaptive immune response.[5] However research has shown that Batrachochytrium dendrobatidis suppresses the mucosal antibody response by releasing factors that inhibit lymphocyte proliferation by induction of apoptosis.[18]
While all post-metamorphic amphibians can experience parasitization by Batrachochytrium dendrobatidis, morbidity and mortality vary widely among species, populations and individuals.[12] Host tolerance to Batrachochytrium dendrobatidis may be due to differences in innate and adaptive immune mechanisms, proximity to metamorphosis, inter- and intra-specific interactions, or behavioral characteristics.[7]
Newly metamorphosing amphibians are particularly susceptible to infection due to changes in the natural immune system at that time. During metamorphosis, a rapid increase in corticosteroid hormones causes a loss of lymphocytes. The suppression of the adaptive immune system at the same time as the tadpoles' skin becomes keratinized leaves the individual vulnerable to colonization by Batrachochytrium dendrobatidis. This is compounded if the tadpoles’ mouthparts are already infected, emerging zoospores can establish themselves in the newly keratinized skin, rapidly overwhelming the host.[15] Stress responses caused by inter- and intra-specific interactions like competition for resources and breeding can similarly impair adaptive immune function in amphibians.[6]
Species-specific behavioral characteristics including aggregative behaviors, microhabitat selection, or basking may affect the likelihood of infection and disease.[6] Aggregative behaviors like mating or larval schooling can increase proximity to infected individuals and therefore increase the likelihood of infection.[4] Species that have slightly warmer microhabitats or for which basking behavior is common may have decreased pathogen abundance of the heat-intolerant fungus.[8] Environmental variables, in specific temperature, can compound species-specific differences in host susceptibility.[18]
Climate change models predict that anthropogenic induced warming over the last century will soon cause changes in temperature variances and extremes.[16] These fluctuations are likely to alter the relationship between ectotherm hosts and pathogens. In particular the relationship between amphibians and Batrachochytrium dendrobatidis is likely to be impacted. As ectotherms, amphibians invest more energy in immune processes when temperatures are warmer rather than cooler.[7] The abundance of Batrachochytrium dendrobatidis is highly temperature dependent; as temperature decreases, abundance increases; and as temperature increases, abundance decreases. While it is not entirely clear which has the greater magnitude of change— increased growth of Batrachochytrium dendrobatidis at lower temperatures or more effective host-immune response at higher temperatures— they work concurrently to alter the pathogen-host relationship.[18] Amphibians maintained for extended periods of time below their thermal optima have a reduced capacity to fight off Batrachochytrium dendrobatidis infection.[18] As such, even small decreases in temperature may impair immunity.[15]
Organisms at lower trophic levels generally acclimate to changes in temperature faster than those at higher trophic levels, thus the parasitic pathogen may acclimate faster, causing a resulting lag in optimal host immunity.[7] As a result, scientists have hypothesized that temperature fluctuations from cold to warm will increase the susceptibility of amphibians to pathogenic infection, while a fluctuation from warm to cold, decrease susceptibility. This “climate variability” hypothesis has been supported by research on temperature impacts on Batrachochytrium dendrobatidis and host relationships.
While sustained increases in temperature are likely to be beneficial to amphibian hosts of heat-intolerant Batrachochytrium dendrobatidis, extreme short-term variations in temperature may make it challenging to fully access the potential consequences of climate change. Acute changes in temperature may persist long enough to cause thermal stress in the host but are too short to be therapeutic in ridding the host of infection or to allow for thermal acclimation.[16]
Furthermore, Batrachochytrium dendrobatidis has evolved mechanisms to limit host behaviors that are detrimental to its performance. In ectotherms, basking can generate a behavioral fever, increasing body temperature. Because elevated temperatures may decrease the parasitic load by limiting parasitic performance and promoting immune function, it is in the best interest of the fungus to limit this behavior. At high infection levels Batrachochytrium dendrobatidis can increase the heat sensitivity of the host, reducing the critical thermal maxima of hosts by up to ~4°C, effectively discouraging the basking behavior. The parasitic fungus manipulates host physiology by lowering the thermal tolerance of the amphibian, promoting movement to a cooler microhabitat that favors the parasite. This adaptive manipulation of host behavior by the fungus functions to increase parasitic performance through changes in the host's behavior. Predicted increases in future global climate compounded with a diminished upper temperature threshold caused by the fungus, could be hazardous to amphibian hosts as conditions move away from optimal conditions and closer to the lethal critical maxima. This is further exacerbated as altered climate patterns may lead to subsequent changes in phenology, resource availability, and inter- and intra-species interactions that threaten survival.[16]
Geographic Distribution
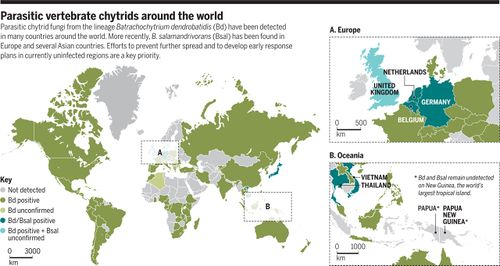
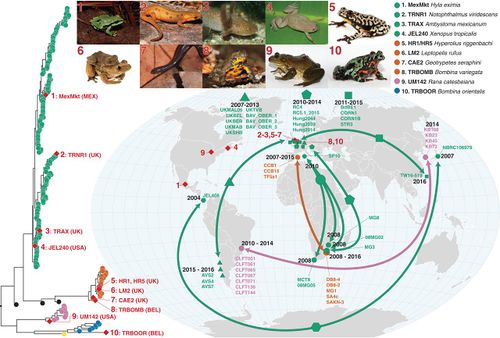
Historically it was widely believed that Batrachochytrium dendrobatidis had originated in Africa. Skin samples from African clawed frogs (Xenopus laevis) collected in 1938 were the earliest known evidence of Batrachochytrium dendrobatidis. This led researchers to believe that the fungus had originated in Africa where it was then spread through the international trade of African clawed frogs, which for decades were used in human pregnancy tests.[6]
It was expected that amphibian populations at the origin of disease would have had time to evolve stable host-pathogen dynamics and have relative resistance to the pathogen.[21] Xenopus laevis is relatively resistant to the fungus, and acts as a natural carrier, because despite having no clinical signs, infected individuals carry light infections.[1] Xenopus laevis then spread the pathogen to other natural carriers, notably the American bullfrog (Rana catesbeiana), which were internationally traded in the millions as a food item. The original vector of disease for this pathogen was likely amphibians native to the area in which Batrachochytrium dendrobatidis is endemic that were exported for food, research, private collection, or even just accidently removed from their environment (Fig 5).[5]
While the international and intranational trade of amphibians certainly played a role in spreading the pathogen, new research suggests that Batrachochytrium dendrobatidis originated in East Asia, with its emergence dating back to the early 20th century. Phylogenetic analysis of 234 isolates pointed to one lineage (BdASIA-1), originating in the Korean peninsula, as being the ancestral population. By using the mutation rate of Batrachochytrium dendrobatidis’ nuclear and mitochondrial DNA, the BdASIA-1 lineage was estimated to have emerged approximately 120 to 150 years ago.[20] The complete lack of chytrid-related population decline in eastern Asia, despite the widespread presence of the fungus, is evident of stable host-pathogen dynamics.[5]
The commercial trade of amphibians is still continuing to transmit the fungus globally (Fig 4).[20] All known lineages of Batrachochytrium dendrobatidis have been found in globally traded amphibians (Fig 5). This is a grave concern for not only amphibians but for salamanders, as well, as there is potential for the deadly spread of the newly emerged, Batrachochytrium salamandrivorans.[19] The global dissemination of amphibian chytrid fungus needs to be addressed and mitigated through policy and legislation to protect the biodiversity in the few remaining areas where the fungus has not yet been detected.[20] According to climate predictions, Batrachochytrium dendrobatidis is projected to increase its range, potentially resulting in more amphibian populations coming in contact with the pathogen and further complicating the issue.[7]
Conclusion
There is still a significant amount of research necessary before any real reversal can occur in amphibian population decline. In order to understand and create plans to mitigate future loss of amphibian populations, a deeper understanding of how host-parasite dynamics are shifted based on temperature and other environmental factors is necessary.[8]
Unfortunately, there are very few therapeutic treatments that can be done to reverse infection. Antifungal treatments of Batrachochytrium dendrobatidis cultures were highly effective in eradicating the fungus. However, Batrachochytrium dendrobatidis is far less susceptible to topical antifungal treatment when present in a host. Increased sloughing of epidermal cells causes multiple layers of keratinized cells to build up, which combined with changes to the cell structure reduces permeation by the antifungal drugs.[1] Another potential therapy is to increase the effectiveness of the innate immune system by experimentally exposing amphibians to the symbiotic bacteria, Janthinobacterium lividum. This symbiotic bacteria produces antifungal compounds that target chytrid fungus.[17]
Crucial understanding of how Batrachochytrium dendrobatidis will continue to spread and the potential of susceptible host populations to evolve resistance to the fungus may come from further analysis of the relationship between the pathogen and resistant amphibian species in Asia where the pathogen originated.
References
- ↑ 1.00 1.01 1.02 1.03 1.04 1.05 1.06 1.07 1.08 1.09 1.10 1.11 1.12 1.13 1.14 1.15 Berger et al. 2005. Life cycle stages of the amphibian chytrid Batrachochytrium dendrobatidis. Inter-Research. 68:52-63
- ↑ 2.0 2.1 2.2 2.3 Weldon et al. 2004. Origin of the Amphibian Chytrid Fungus. Emerging Infectious Diseases. 10(12):2100-2105
- ↑ Berger et al. 1998. Chytridiomycosis causes amphibian mortality associated with population declines in the rain forests of Australia and Central America. PNAS. 95:9031-9036
- ↑ 4.0 4.1 4.2 4.3 4.4 4.5 4.6 Piotrowski et al. 2004. Physiology of Batrachochytrium dendrobatidis, a Chytrid Pathogen of Amphibians. Mycologia. 96:9-15
- ↑ 5.0 5.1 5.2 5.3 5.4 Fisher, M.C., T.W.J. Garner. 2020. Chytrid fungi and global amphibian declines. Nature Reviews Microbiology. (1740-1526).
- ↑ 6.00 6.01 6.02 6.03 6.04 6.05 6.06 6.07 6.08 6.09 6.10 6.11 Rosenblum et al. 2010. The Deadly Chytrid Fungus: A Story of an Emerging Pathogen. PLoS Pathogens. 6(1):e1000550
- ↑ 7.0 7.1 7.2 7.3 7.4 7.5 7.6 7.7 7.8 7.9 Bradley et al. 2019. Shifts in temperature influence how Batrachochytrium dendrobatidis infects amphibian larvae. Health and Medicine. 14:(9)
- ↑ 8.0 8.1 8.2 8.3 Stevenson et al. 2013. Variation in Thermal Performance of a Widespread Pathogen, the Amphibian Chytrid Fungus Batrachochytrium dendrobatidis. PLoS ONE. 8(9):e73830
- ↑ Rooij et al. 2015. Amphibian chytridiomycosis: A review with focus on fungus-host interactions. Veterinary Research. 46(1):137.
- ↑ 10.0 10.1 10.2 Longcore et al. 1999. Batrachochytrium Dendrobatidis gen. et sp. nov., a Chytrid Pathogenic to Amphibians. Mycologia. 91:219-227
- ↑ Margulis and Chapman. 2009. Chytridiomycota. In: Kingdoms and Domains: An Illustrated Guide to the Phyla of Life on Earth. Academic Press, Cambridge, pp.220-221.
- ↑ 12.0 12.1 12.2 12.3 12.4 12.5 12.6 12.7 Greenspan et al. 2012. Host invasion by Batrachochytrium dendrobatidis: fungal and epidermal ultrastructure in model anurans. Dis Aquat Org. 100:201-210
- ↑ Muletz et al. 2014. Unexpected Rarity of the Pathogen Batrachochytrium dendrobatidis in Appalachian Plethodon Salamanders: 1957–2011. PLOS. 9:e103728
- ↑ 14.0 14.1 Nichols et al. 2001. EXPERIMENTAL TRANSMISSION OF CUTANEOUS CHYTRIDIOMYCOSIS IN DENDROBATID FROGS. BioOne. 37(1):1-11
- ↑ 15.0 15.1 15.2 15.3 15.4 15.5 15.6 Rollins-Smith et al. 2011. Amphibian Immune Defenses against Chytridiomycosis: Impacts of Changing Environments. Integrative and Comparative Biology. 51(4):552-562
- ↑ 16.0 16.1 16.2 16.3 Greenspan et al. 2017. Infection increases vulnerability to climate change via effects on host thermal tolerance. Scientific Reports. 7:9349
- ↑ 17.0 17.1 Walke et al. 2015. Community Structure and Function of Amphibian Skin Microbes: An Experiment with Bullfrogs Exposed to a Chytrid Fungus. PLoS ONE. 10(10):e0139848
- ↑ 18.0 18.1 18.2 18.3 Ribas et al. 2009. Expression Profiling the Temperature-Dependent Amphibian Response to Infection by Batrachochytrium dendrobatidis. PLoS ONE. 4(12):e8408
- ↑ 19.0 19.1 Bower et al. 2017. Amphibians on the brink: Preemptive policies can protect amphibians from devastating fungal diseases. Science. 357:454-455
- ↑ 20.0 20.1 20.2 20.3 O’Hanlon et al. 2018. Recent Asian origin of chytrid fungi causing global amphibian declines. Science. 360:621-627
- ↑ Becker et al. 2017. Variation in phenotype and virulence among enzootic and panzootic amphibian chytrid lineages. Elsevier. 26:45-50
Authored for BIOL 238 Microbiology, taught by Joan Slonczewski, 2018, Kenyon College.