Treponema Pallidum: Genome, History of Infection, and Public Health
Introduction
Treponema Pallidum: Genome, History of Infection, and Public Health
by Katie Ceniza-Levine
Human treponemal diseases, including the sexually transmitted infection syphilis, have existed as early as the fifteenth century, though the exact origins of syphilis are debated. The Columbian hypothesis states that Christopher Columbus and his crew brought syphilis to Europe after he returned from his voyage to the New World in the late fifteenth century. In the pre-Columbian hypothesis, syphilis, and other treponemal infections such as yaws, were already in Europe and across the world before Columbus' time– but they were interpreted as leprosy, not a treponemal infection. [1] In the early twentieth century, two scientists named Schaudinn and Hoffman identified the bacterium that causes syphilis, and they named it Treponema Pallidum.[1] Although syphilis rates can be mitigated with penicillin, cases persist in the twenty-first century.
Genome
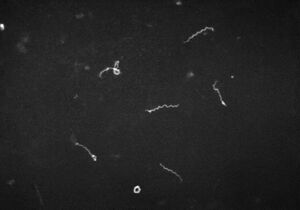
Treponema Pallidum
Phylum: Spirochaets
Order: Spirochaetaceae[1]
Subspecies and the human pathogen it causes:
- Pallidum: syphilis
- Endemicum: betel (endemic syphilis)
- Carateum: pinta
- Pertenue: yaws[3]
Genome sequencing
T. pallidum cannot be visualized by bright-field microscopy, and scientists have had difficulties culturing it in a lab. For this reason, much of our information on T. pallidum comes from dark-field, fluorescence, and electron microscopy, genome sequencing.[4] The genome of the Nichols strain of T. pallidum was sequenced in 1998, and then resequenced in 2013.[5][6] The results showed that T. pallidum has a circular chromosome of approximately 1,139,000 base pairs and over one thousand open reading frames. The average gene length of each gene is 1,023 base pairs.[7] The genome of T. pallidum is smaller compared to other prokaryotic genomes– it has only a fourth of the base pairs of E. Coli.[5] The T. pallidum genome is small because it has evolved to stop producing enzymes for the tricarboxylic TCA cycle. The TCA cycle converts sugar into water and carbon dioxide. Since T. pallidum does not have that ability, it relies on a host for amino acid production.[4]
Metabolism
T. pallidum’s ability to synthesize its own materials is limited; its genome does not have many of the enzymes required for biosynthesis.[5][7]
Therefore, it relies on transporter proteins that can take nutrients from its host and environment. 5% of T. pallidum's ORFs code for eighteen transporters.[5] Survival outside of the host is very limited. T. pallidum is capable of glycolysis to produce ATP, though its glycolytic pathway is slightly modified from other bacteria. T. pallidum uses a phosphofructokinase instead of an ATP-dependent enzyme– which saves T. pallidum ATP. [8]
In vitro fertilization
For almost a century, researchers struggled to cultivate T. pallidum in vitro. Long term in vitro was finally achieved in 2017. The Nichols strain of T. pallidum can be cultured in vitro indefinitely in rabbits, or co-cultured in vitro with rabbit cells, provided it is supplied with the right amount of oxygen and carbon dioxide.[9] After examining the genome of T. pallidum in rabbits compared to its genome in vitro, researchers found very minimal changes to the overall genome. However, transcription levels varied significantly, suggesting that T. pallidum can react to its surroundings in limited contexts. [10]. Notably, transporter proteins and flagellar proteins were expressed at similar levels in vitro and in rabbits, suggesting that such capabilities are not affected by the external environment.[10] T. pallidum is able to change gene description to adjust to its environment, because it has four alternative sigma factors.[8] More recently, scientists have been able to culture T. pallidum directly from infected persons, using probes that separate the pathogen's DNA from the host genome. Such techniques do not require in vitro or rabbit propagation for sequencing.[11]
Gene expression
Gene expression varies widely across the chromosome, but transcription was found to be highest in an area responsible for a ribosomal protein operon.[7] Other genes that were highly expressed in T. pallidum included genes for proteins associated with metabolism, motility, and the cell envelope.[7][10] Such genes are part of what allows T. pallidum to be highly virulent. One notable facet of T. pallidum is that it has an irregular heat shock response system. Although it has some heat shock proteins, it lacks the gene that transcribes them. It does not have sigma factor σ32, which explains why T. pallidum is sensitive to heat [5][8].
Virulence factors
The outer membrane is a major factor that contributes to T. pallidum's virulence. Its outer membrane lacks lipopolysaccharide (LPS), which is often found in gram-negative bacteria. The lipoproteins that T. pallidum does have are often below the surface of the cell.[8] Furthermore, T. pallidum does not have many pathogen-associated molecular patterns (PAMPs) on its surface.[8] TprK allows the few outer membrane proteins of its genome to have varying antigens. For these reasons, T. pallidum can repeatedly enter and infect a host multiple times before being identified as a threat.[3]
There are other attributes of T. pallidum that make it virulent. It has a flexible, flat-wave morphology that allows it to more easily penetrate and go deep into the tissue. This increases the space and opportunities that T. pallidum has to infect the host.[8] Adherence to the host cell and binding to the extracellular matrix is necessary for transmission. T. pallidum is able to complete that function because it has many adhesins, including fibronectin-binding proteins, laminin-binding proteins, and BamA, which puts outer membrane proteins into the outer membrane.[8][3] Finally, T. pallidum is very motile, and that is what allows it to enter and infect host cells.[3] The Nichols strain genome has thirty-six genes relating to flagellar structure and function, expressed across four different operons.[5]
Infection and Treatment
Transmission and Stages of Infection


Acquired/Venereal syphilis: Spreads through sexual intercourse if one makes direct contact with an infected, active lesion.[12]
Congenital syphilis: Syphilis can be transmitted through the placenta. If a pregnant person has syphilis then they can pass on the infection to their child, and that child may be born with either secondary or tertiary syphilis.[3]
Primary syphilis: This period lasts about 2-6 weeks and is associated with a chancre– an ulcer at the site of the infection. It is small (about a center in diameter), hard and painless.[4][12] After a few weeks it will heal and scar over, because the macrophages cause the treponemas to undergo phagocytosis.[12] Once the chancre has healed, the syphilis is considered to be in a latent stage. The infected person may be asymptomatic for up to five years.[4]
Secondary syphilis: defined by a rash over the whole body, particularly around the palms of the hand and feet; the rash will eventually crust over. The which rash resembles the rashes of other illnesses (giving syphilis its nickname as the “great imitator.”)[4] Other symptoms of secondary syphilis include pyrexia, body pains, fatigue, fever and sore throat. At this point, the patient can still respond to antibiotics. If untreated, 70% of infected persons will enter the latent period; the other 30% will progress to tertiary syphilis.[3]
Tertiary syphilis: This stage is associated with cardiovascular symptoms, such as aneurysms, and neurological symptoms, such as seizures, blindness, and meningitis. At this stage the patient may have gummas– necrotic granulomatous lesions– on their skin and bones.[3] In some cases the patient may develop syphilis and die.[4]
Latent stage: The infected person is asymptomatic but can still test positive on serological tests. The latent stage is considered early within one year of infection, and it is considered late past one year of infection.[12]
Diagnosis and Screening
Direct detection: To diagnose patients with syphilis, doctors will often use dark-field microscopy to identify the presence of T. pallidum directly.[12] The method is fairly effective, though there are limits. The accuracy of the diagnosis depends on the lesions and the skill of the person studying the microscopy, and there are certain areas of the body that dark-field microscopy cannot examine.[12] Direct fluorescent antibody tests and nucleic-acid amplification tests can also be used to detect T. pallidum, but they are more often used in research labs.[12]
Serological Test for Syphilis (STS): Such tests are affordable, easy to obtain, and give results quickly. However, they are known for false positives and are not sensitive to primary or tertiary syphilis.[12] For this reason, it is common practice in the U.S. to use multiple STSs to confirm a syphilis diagnosis– one non-treponemal test and one treponemal STS.[12]
History of Treatment

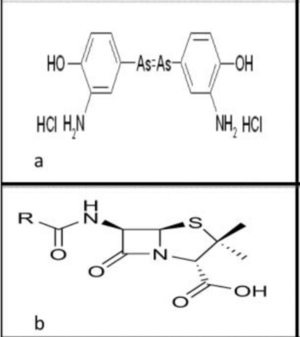
15th and 16th century: Earlier treatments were meant to act as purgatives to get rid of the “bad blood” in the patient. New World plants were popular treatments– particularly the guanic tree, which was recommended by the priest Ulrich von Hutten. It was boiled and taken as a drink, which the patient would take for thirty days.[1] Another treatment was mercury, which was recommended by Paracelsus. Mercury could be taken as a pill or applied directly on the patient’s body. Mercury was administered in doses that were high enough to be considered toxic.[1] Both of the treatments listed were popular because it induced diarrhea and sweating. Bismuth salts would later replace mercury as a less toxic alternative in the late nineteenth century.[1]
Early 20th Century: In the early twentieth century, Paul Ehlrich and his lab attempted to find a “magic bullet” – a cure that could hurt the pathogen without affecting the host cell (the concept is now known as selective toxicity.)[4] Elrich and his lab tested several compounds, for which he received the Nobel Prize in 1908.[1] In 1909, his student, Sahachiro Hata, was testing arsenic compounds specifically when he identified arsphenamine. It was the 606th compound the lab tested, so it was known as Ehlrich 606, or sometimes Salvarsan– and it was one of the most widely used treatment against syphilis until the 1940s.[14] Later, another arsenic compound and drug was invented around that time, known as Neosalvarsan. Compared to Salvarsan it was less toxic and dissolved more easily in water.[1] Inducing a fever was also a popular syphilis treatment at the time. These methods included turpentine, tuberculine, mercury, and Salmonella typhi. In 1917, Julis Wagner-Jauregg used malaria to induce fevers in his patients, then would control the symptoms with quanine. He received the Nobel Prize in 1927.[1]
Although it was known that Salvarsan was an arsenic compound, its exact composition was debated and questioned for decades.[13] Ehlrich’s initial compound was a reaction between 3-nitro-4-hydroxyphenyl-arsonic acid with dithionite. NO2 was reduced to NH2, and ASv was reduced to ASi, forming: 3-H2N-4-HOC6H3As. However, that compound was not consistently reproducible; different batches had varying levels of toxicity.[13] Ehlrich initially believed that the free base of the compound was in Structure 1. However, in 2005, electrospray ionization mass spectrometry (ESI spectrometry) revealed that the free base was actually in Structure 2– which includes 3 As-R bonds, or Structure 3, which includes 5 As-R bonds.[13]
Penicillin: The antibiotic properties of penicillin were known as early as 1896, when a French medical student, Ernest Duchesne, injected mold into guinea pigs with typhoid fever bacillus.[4] Then in 1928, Alexander Fleming rediscovered the antibiotic uses of penicillin in his lab. While he washed old petri dishes that had been used to culture Staphylococcus aureus, he noticed that one petri dish had mold on it. Where there was mold, S. aureus would not grow. The mold belonged to the penicillin family. By 1943, penicillin was the main treatment of syphilis.[14]
Penicillin is made from cytosine and valine, which condense to form a beta-lactam ring structure. That structure is similar to a part of the structure of peptidoglycan, which allows it to target transpeptidase and other proteins used for cell wall production.[4] Transpeptidase allows peptidoglycan to bond and form the cell wall. Penicillin also activates proteins that hydrolyze peptidoglycan; as the cell grows, the cell wall will not be able to withstand the pressure, causing the cell to burst.[4]
Public Health in the United States
Syphilis has been associated with xenophobia and race since the sixteenth century. Wherever it appeared, the neighboring country was blamed. Before its official identification, it was referred to as the French disease, the Neapolitan disease, the Polish disease, the German disease, the Spanish/Castilian disease, and the Christian disease. In India, the Hindus and Muslims blamed each other for the spread of syphilis, before attributing it to Europeans. [1] In the twentieth and twenty-first centuries, the historical background and stigma surrounding syphilis later gave way to issues with eugenics and racial health disparities.
The Tuskegee Experiment was an experiment in which Black men were told that they were being treated for “bad blood," a vague term that, at the time, could refer to a myriad of illnesses.[15] The men actually had syphilis, and they were not given proper treatment, but instead were given placebos and examined so that doctors could see the progression of syphilis. The experiment lasted for forty years, and many participants died.[15] President Clinton apologized for the atrocities in 1997, but the ramifications persist today.[15] In the United States there are racial disparities in syphilis and other STIs, and the experiment exacerbated a deep-rooted distrust of health officials in the African-American community.[16][4]
Incidence of Syphilis in the 20th Century
In the year 1940, 458,4000 cases of syphilis were reported.[17] To mitigate the spread of venereal diseases, diagnostic facilities were improved and expanded. Syphilis was tested more frequently, and after the introduction of penicillin, many cases were treatable. The United States Public Health Service created programs to stop the spread of venereal disease, and their treatment efforts were funded by the Lanham Act. Education about syphilis also increased throughout the country.[17] That progress eventually slowed. Blood tests were administered less frequently, syphilis was dropped from the curriculum in some medical schools, and the federal government cut the funding for venereal disease prevention to only $3,000,000.[18]
Cases doubled by the 1960s, and the U.S. Public Health service created a task force to manage the situation. The task force concluded that the known mitigation measures were not utilized, and called for more funding and resources.[18] Another potential reason for that rise of cases is that birth control pills were becoming increasingly popular, and such pills would not manage the spread of STIs. Cases eventually decreased, until the 1980s, when public health efforts focused more on the HIV and AIDS epidemic.[19]
One of the most notable government, public health initiatives to manage syphilis rates was the National Campaign to Eliminate Syphilis (SEP) which began in 1999. By that time, it was relatively easy to diagnose and treat syphilis. The campaign was meant to be a public health intervention collaboration between the CDC and many different agencies.[16] The project utilized five different intervention strategies: enhanced surveillance and outbreak response, community involvement and organizational partnerships, enhanced health promotion, expanded clinical and laboratory services, and partner services.[16]
Syphilis rates in the United States also rose in 2020, during the COVID-19 pandemic. In 2020, seven million people across the world were infected with syphilis. In the United States, active cases of syphilis rose from 129,000 to almost 134,000.[20] This rise in cases is due to a variety of factors, mainly involving the COVID-19 pandemic. Resources, including available workers and funding, were reallocated from STI prevention to COVID-19 efforts. 83% of STI programs were unable to continue their work during COVID-19.[20] This reduction in programming made it more difficult for people to screen for STIs. After the pandemic, syphilis tests decreased by 3% per month in the emergency department.[21] The problems became so severe that the United States enacted a five year plan to reverse the surges in STI rates, known as the Sexual Transmitted Infections National Strategic Plan.[20] Researchers are studying Tpr genes in the treponema pallidum genome to find a vaccine.[11]
Public Health Disparities
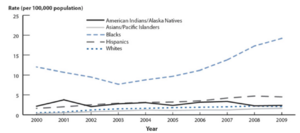
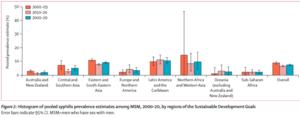
Race: In the 1990s, at the beginning of SEP, Black Americans were disproportionately affected by the illness– making up 80% of the cases of primary and secondary stage syphilis.[16] Despite the program’s efforts, cases began to rise again beginning in 2001. The rates of STIs in Black populations are significantly higher compared to other groups, and for several reasons. Black Americans are more likely to face socioeconomic issues, which can influence healthcare experiences, including STIs. It is more difficult for lower income people to have access to the healthcare systems to manage and prevent STIs.[16] Furthermore, the patterns of sexual intercourse amongst the Black populations of the U.S. can also contribute to higher cases. Since cases of STI’s are already high amongst the Black community, and since people within the Black community are more likely to have other Black partners, that increases the rates of incidence with STI’s.[16]
LGBT: Rates of syphilis have been steadily increasing amongst men who have sex with men (MSM) in the United States. In 2000, it was estimated that 7% of primary and secondary syphilis cases were transferred between MSM. In 2004, that number rose to 64%, by 2015, the estimate was at 81.7%. Such rates are higher than the rate of syphilis amongst women, and men who only have sex with men.[19][23] This is true across most states, including states with low incidence of syphilis– showing that syphilis disproportionately affects MSM across the United States. The states with the highest rate of incidence among MSM include Arizona, Louisiana, Mississippi, Nevada, and North Carolina.[23]
The higher incidence rate of syphilis in MSM is true in countries outside of the United States as well. From 2000-2009, the incidence of syphilis worldwide amongst MSM was estimated to be 8.9%, and it decreased to 6.6% from 2010-2020, creating an average incidence rate of 7.5% across those two decades.[22] Three regions– Northern Africa and Western Asia, Eastern and South-Eastern Asia, and Latin America and the Caribbean– had higher incidence rates amongst MSM compared to the rest of the globe.[22]
Conclusion
Treponemal infections and diseases have been around for decades, but more research is being done everyday to understand more about this opportunistic spirochete and mitigate infection rates.
References
- ↑ 1.0 1.1 1.2 1.3 1.4 1.5 1.6 1.7 1.8 1.9 M., Sarbu, I., Matei, C., Benea, V., & Georgescu, S. R. (2014). Brief history of syphilis. Journal of medicine and life, 7(1), 4.
- ↑ 1961. Schwartz, W.F.[Format.CDC.]]
- ↑ 3.0 3.1 3.2 3.3 3.4 3.5 3.6 3.7 3.8 D. I., & Johnson, D. I. (2018). Treponema spp. Bacterial Pathogens and Their Virulence Factors, 381-387..
- ↑ 4.00 4.01 4.02 4.03 4.04 4.05 4.06 4.07 4.08 4.09 4.10 [Slonczewski, J. L., & Foster, J. W. (2020). Microbiology: An evolving science: Fifth Edition. WW Norton & Company.]
- ↑ 5.0 5.1 5.2 5.3 5.4 5.5 Fraser, C. M., Norris, S. J., Weinstock, G. M., White, O., Sutton, G. G., Dodson, R., ... & Venter, J. C. (1998). Complete genome sequence of Treponema pallidum, the syphilis spirochete. Science, 281(5375), 375-388.
- ↑ Pětrošová, H., Pospíšilová, P., Strouhal, M., Čejková, D., Zobaníková, M., Mikalova, L., ... & Šmajs, D. (2013). Resequencing of T. pallidum ssp. pallidum strains Nichols and SS14: correction of sequencing errors resulted in increased separation of syphilis treponeme subclusters. PloS one, 8(9), e74319.
- ↑ 7.0 7.1 7.2 7.3 Šmajs, D., McKevitt, M., Howell, J. K., Norris, S. J., Cai, W. W., Palzkill, T., & Weinstock, G. M. (2005). Transcriptome of Treponema pallidum: gene expression profile during experimental rabbit infection. Journal of bacteriology, 187(5), 1866-1874.
- ↑ 8.0 8.1 8.2 8.3 8.4 8.5 8.6 Radolf, J. D., Deka, R. K., Anand, A., Šmajs, D., Norgard, M. V., & Yang, X. F. (2016). Treponema pallidum, the syphilis spirochete: making a living as a stealth pathogen. Nature Reviews Microbiology, 14(12), 744-759.
- ↑ Edmondson, D. G., & Norris, S. J. (2021). In vitro cultivation of the syphilis spirochete Treponema pallidum. Current Protocols, 1(2), e44.
- ↑ 10.0 10.1 10.2 De Lay, B. D., Cameron, T. A., De Lay, N. R., Norris, S. J., & Edmondson, D. G. (2021). Comparison of transcriptional profiles of Treponema pallidum during experimental infection of rabbits and in vitro culture: Highly similar, yet different. PLoS Pathogens, 17(9), e1009949.
- ↑ 11.0 11.1 Giacani, L. (2022). Strategies for syphilis vaccine development. Brazilian Journal of Sexually Transmitted Diseases, 34.
- ↑ 12.0 12.1 12.2 12.3 12.4 12.5 12.6 12.7 12.8 Stamm, L. V. (2015). Syphilis: antibiotic treatment and resistance. Epidemiology & Infection, 143(8), 1567-1574.
- ↑ 13.0 13.1 13.2 13.3 Lloyd, N. C., Morgan, H. W., Nicholson, B. K., & Ronimus, R. S. (2005). The composition of Ehrlich's salvarsan: resolution of a century‐old debate. Angewandte Chemie International Edition, 44(6), 941-944.
- ↑ 14.0 14.1 14.2 Zaffiri, L., Gardner, J., & Toledo-Pereyra, L. H. (2012). History of antibiotics. From salvarsan to cephalosporins. Journal of Investigative Surgery, 25(2), 67-77.
- ↑ 15.0 15.1 15.2 [Tobin, M. J. (2022). Fiftieth anniversary of uncovering the tuskegee syphilis study: the story and timeless lessons. American Journal of Respiratory and Critical Care Medicine, 205(10), 1145-1158. Tobin, M. J. (2022). Fiftieth anniversary of uncovering the tuskegee syphilis study: the story and timeless lessons. American Journal of Respiratory and Critical Care Medicine, 205(10), 1145-1158.]
- ↑ 16.0 16.1 16.2 16.3 16.4 16.5 16.6 Valentine, J. A., & DeLisle, S. J. (2012). Reducing disparities in sexual health: lessons learned from the campaign to eliminate infectious syphilis from the United States. In The new public health and STD/HIV prevention: Personal, public and health systems approaches (pp. 361-382). New York, NY: Springer New York.
- ↑ 17.0 17.1 Rosenthal, T. (1944). Venereal Disease in Wartime. The American Journal of Nursing, 44(2), 104–106.
- ↑ 18.0 18.1 Rosenblum, B. F. (1964). Steps in the eradication of syphilis. Journal of the National Medical Association, 56(3), 252.
- ↑ 19.0 19.1 Shockman, S., Buescher, L. S., & Stone, S. P. (2014). Syphilis in the United States. Clinics in Dermatology, 32(2), 213-218.
- ↑ 20.0 20.1 20.2 Nazir, A., Masood, W., Ahmad, S., Nair, A. M., Aborode, A. T., Khan, H. D., ... & Audah, K. A. (2022). Rise of syphilis surge amidst COVID-19 pandemic in the USA: a neglected concern. Annals of Medicine and Surgery, 80, 104239.
- ↑ Stanford, K. A., Almirol, E., Schneider, J., & Hazra, A. (2021). Rising syphilis rates during the COVID-19 pandemic. Sexually Transmitted Diseases, 48(6), e81-e83.
- ↑ 22.0 22.1 22.2 Tsuboi, M., Evans, J., Davies, E. P., Rowley, J., Korenromp, E. L., Clayton, T., ... & Chico, R. M. (2021). Prevalence of syphilis among men who have sex with men: a global systematic review and meta-analysis from 2000–20. The Lancet Global Health, 9(8), e1110-e1118.
- ↑ 23.0 23.1 de Voux, A., Kidd, S., Grey, J. A., Rosenberg, E. S., Gift, T. L., Weinstock, H., & Bernstein, K. T. (2017). State-specific rates of primary and secondary syphilis among men who have sex with men—United States, 2015. Morbidity and Mortality Weekly Report, 66(13), 349.
Authored for BIOL 238 Microbiology, taught by Joan Slonczewski, 2023, Kenyon College