Anellovirus: Difference between revisions
(103 intermediate revisions by the same user not shown) | |||
Line 1: | Line 1: | ||
==Introduction== | ==Introduction== | ||
[[Image:Anellotree.png|thumb|300px|right|Phylogenetic tree of known human anelloviruses using ORF1 sequences from GenBank [[#References|[17]]].]] | [[Image:Anellotree.png|thumb|300px|right|Figure 1. Phylogenetic tree of known human anelloviruses using ORF1 sequences from GenBank [https://link.springer.com/article/10.1007%2Fs00705-015-2363-9 (Spandole et al. 2015)] [[#References|[17]]].]] | ||
<br>By Julia Josowitz<br> | <br>By Julia Josowitz<br> | ||
<br> | <br> What are anelloviruses and what is our current understanding of their interactions with humans? <br> | ||
<br | |||
<br>Anelloviruses are small, circular viruses found in blood plasma. They are single stranded and negative-sense DNA viruses, which means that its DNA can be directly transcribed into mRNA, as its DNA is the "template" strand. While they are not known to cause any harm, high viral loads are associated with immune suppression and diseases that correlate with immune suppression, such as hepatitis, cancer, and autoimmune diseases [[#References|[3]]]. The first anellovirus discovered was torque teno virus (TTV) in 1997, which was initially believed to be part of the pathology of hepatitis, but since then these viruses have been found to be relatively widespread and heterogeneous, with no known pathogenicity [[#References|[14]]][[#References|[17]]]. TTV is still used as the prototypical anellovirus for many studies today, and it is one of the most prevalent. Since their discovery, it has become clear that these viruses are very ubiquitous, representing about 70% of the viruses found within human blood and tissues [[#References|[9]]]. <br> | |||
<br> <br> | <br>Within the family <i> Anelloviridae</i> there are twelve known genera, but originally there were thought to be three prototypical genera: <i> Alphatorquevirus</i>, <i>Betatorquevirus</i>, and <i> Gammatorquevirus</i>. <i>Alphatorquevirus</i> includes TTV, while <i>Betatorquevirus</i> includes torque teno mini virus (TTMV) and <i>Gammatorquevirus</i> includes torque teno midi virus (TTMDV). The high mutation rates and diversity have led to high-throughput sequencing categorizing the virus into even more genera [[#References|[4]]]. These viruses have also been found in cats, dogs, and even pigs and bats. In humans, these viruses are very prevalent, with more than 80% of the population infected, and they appear to establish persistent infections that remain within the body for the rest of the human life. As shown in Figure 1, they are also very diverse, with at least 41 different species infecting humans as of 2012 [[#References|[6]]]. Despite this diversity, TTV-8 is currently thought to be the most prevalent anellovirus in humans [[#References|[8]]]. Although these viruses remain in the body after infection and infect such a large part of the population, their mechanisms of evading the host immune system are unknown. However, their ability to avoid immune clearance and their prevalence suggest an almost commensal relationship with the host. <br> | ||
<br> Anelloviruses are unenveloped viruses that have a capsid made of 12 pentameric capsomers. With microscopy, it appears to be a spherical particle and it has icosahedral symmetry typical of many viruses [[#References|[12]]]. There is no evidence of a lipid envelope, which usually includes proteins that the virus uses to bind host cells. Additionally, nonenveloped viruses tend to be more stable, which likely contributes to the persistence of these viruses within the body. Virion particle sizes vary between genera, with a range from 30 to 50 nm for TTV, but less than 30 nm for TTMV. Anelloviruses are currently unable to be grown <i>in vitro</i> due to lack of compatible cells systems, so the crystal structure of TTV has not been solved and much is unknown about the life cycle of these viruses. It is believed that they replicate within the bone marrow and liver of humans because double stranded replicative intermediates have been found in these tissues, indicating that the single-stranded virus is using the host machinery to replicate there. Anelloviruses have also been found to reside within peripheral blood mononuclear cells, and recent studies have suggested that under certain conditions, the virus may also be able to replicate within these cells, as double stranded intermediates have been found [[#References|[18]]]. <br> | |||
<br>Anelloviruses are | |||
==Transmission and correlation with disease== | |||
<br> While the main transmission route is believed to be blood, there is evidence of multiple other routes. For example, anelloviruses are present in pharyngeal mucus, which suggests that they can also be disseminated via coughing or sneezing. This could help explain how they are so prevalent, as the virus could spread via droplets. Anelloviruses have also been found in the fecal matter of infected organisms and have been detected in sewage water, in addition to some meat products, which suggests an oral route of transmission. These viruses have been found in high numbers in the cervical epithelium, suggesting that they might be transmitted through sexual contact. It is possible that viruses are transmitted from mother to offspring, as the viruses have been found in amniotic fluid and umbilical cord blood, and the newborn viral genotype usually resembles the mother. This could also help explain the prevalence of the viruses in populations, as the virus would consistently be transferred from mother to child. However, it is also possible that newborns become infected early in life simply through contact with the mother [[#References|[17]]]. Finally, because these viruses are prevalent in both animals and humans, it has been suggested that new genera of anelloviruses could arise due to anthropozoonosis, the transmission of pathogens from humans to animals or vice versa, introducing another source of transmission [[#References|[4]]].<br> | |||
<br> | <br> In addition to the prevalence of these viruses in the human population, they have been found to correlate with many diseases, such as hepatitis, HIV, cancer, asthma, and other autoimmune disorders. Patients with both hepatitis and TTV have higher mortality rates than those with hepatitis alone, but to date there is no evidence that the anellovirus contributes to the disease progression. Despite the higher mortality rates, there is no histopathological evidence that co-infection with TTV affects the liver in a different way than hepatitis alone. While TTV has also been detected in neoplastic tissues, it is unclear whether it contributes to cancer progression. Recent research has suggested that the small anellovirus DNA fragments found in human serum could be pathogenic, like geminiviruses in plants, but comprehensive studies of the interactions between the viral DNA and the hosts have yet to be conducted [[#References|[11]]]. Due to the prevalence of these viruses, it is difficult to determine whether they are truly pathogenic and more research must be done to determine their role. <br> | ||
<br> | ==Genome== | ||
<br>The anellovirus genome is made of a circular single stranded DNA molecule that has a GC rich region of 117 nucleotides. Genome size varies based on the host, but the TTV genome ranges from 3.6 to 3.9 kilobases [[#References|[12]]]. Of this, there is a 1.2 kb untranslated region and a 2.6 kb coding region. The untranslated region has been highly conserved, suggesting that it likely plays a role in viral replication. Interestingly, the coding region has two large open reading frames, the first of which encodes a 770 amino acid residue protein and another that encodes a slightly smaller peptide in TTV. These reading frames vary between viral samples. The viral genome produces three viral proteins, shown in Figure 2; the largest viral protein, ORF1, likely encodes the capsid, but the function of the other two is unknown [[#References|[16]]]. Anellovirus is hypothesized to replicate using the rolling-circle method. <br> | |||
[[Image:Manzin et al. genome stx.png|thumb|300px|right|Figure 2. Structure of the anellovirus genome [https://jidc.org/index.php/journal/article/view/26142664/1314 (Manzin et al. 2015)] [[#References|[12]]]. The genome encodes three viral proteins. ORF1 is the largest and most likely encodes the capsid.]] | |||
<br> The genome of anellovirus is very heterogeneous, with high divergence between viral samples. Of the human TTVs in the genus Alphatorquevirus, there were at least 29 known species as of 2015, with more than 40 different genotypes. More specifically, ORF1, which encodes a viral protein, has very high variability with three regions that often experience insertions or deletions, increasing the variability between viruses [[#References|[12]]]. Different genotypes can infect the same host at the same time, even within the same tissue. Researchers hypothesize that this may be due to a high mutation rate in TTV. This is uncommon for DNA viruses, which use the host’s own replicative machinery, making them less error-prone and the chance of mistakes more unlikely. However, research in single stranded DNA viruses such as parvoviruses has found that the single stranded nature of the genome and its ability to encode replication proteins may account for high rates of mutation, as its own replication machinery could be more error-prone. Thus, this could explain the high rates of mutation in anelloviruses. <br> | |||
<br> Recently, it has also been proposed that intra-genomic recombination could account for the heterogeneity of the Anellovirus genome. It has been suggested that TTV has co-evolved with the host for over millions of years, allowing for great variability. However, this same variability makes phylogenetic analysis difficult, as will be discussed later. <br> | |||
<br> Finally, the transmission of these viruses between humans and animals could also contribute to the high variability. <br> | |||
==Potential interactions with the immune system== | |||
<br> Other DNA viruses are known to stimulate immune cells to produce inflammatory cytokines, so Rocchi et al. studied whether TTVs could also have a direct effect on immune system signaling [[#References|[15]]]. More specifically, they tested whether TTV DNA alone could have effects on the immune system. They used lipofectin, which incorporates DNA and protects it from nucleases, to simulate a viral capsid. Spleen cells were treated with lipofectin coupled with TTV DNA, which resulted in an increase in inflammatory cytokines. These results suggest that TTV DNA can directly effect transcription of inflammatory genes within target cells. Inhibitors were used to determine through which receptor TTV DNA was able to change host transcription. Toll-like receptor 9 was found to be the main mediator of the TTV DNA interaction. Toll-like receptor 9 is expressed on phagocytic cells, such as macrophages, as well as antigen-presenting cells, and it binds bacterial and viral DNA. Binding of foreign DNA triggers production of cytokines by the immune cell; thus, through toll-like receptor 9, these results suggest that TTV DNA can directly affect the immune system. <br> | |||
<br> Anelloviruses have also been suggested to modulate the host immune system using micro RNAs (miRNAs). miRNAs are small noncoding RNAs (around 22 nucleotides) that play a role in posttranscriptional gene regulation. Viruses from the herpesvirus and retrovirus families have been found to encode viral miRNAs, and these viruses are also characterized by the ability to establish a persistent infection in their host, much like anelloviruses. It has also been suggested that viral miRNAs may play a role in immune evasion. Kincaid et al. developed computational methods to predict the possibility of miRNAs from the primary sequence of genes [[#References|[10]]]. Their modeling predicted multiple miRNAs encoded by TTVs, and by engineering recombinant TTVs capable of expressing an RNA transgene in addition to the miRNA, they were able to identify a potential target of the viral miRNA, the gene <i>N-myc interactor</i> (<i>NMI</i>). The <i>NMI</i> gene modulates immune signaling, such as cytokines and interferon, which also stimulate the gene through feedback, making it a key regulator of the body's inflammatory response that eliminates pathogens. The authors suggest that the TTV miRNA helps the virus manipulate the immune system by targeting <i>NMI</i> to inhibit interferon and promote immune evasion, as the signaling molecules are suppressed and no immune response is activated. This could help explain how these viruses are so persistent in the human body and avoid immune clearance, as they are able to produce miRNAs that inhibit inflammatory responses of the immune system, which allow them to avoid elimination. However, while all of the human TTVs were found to encode possible miRNAs, none were observed in TTMDVs or TTMVs. <br> | |||
<br> | |||
<br> | <br> Research has also sought to identify the role of the proteins encoded by the viral genome. In a study of swine anelloviruses, it was found that the large viral protein produced by anelloviruses, termed ORF1, down-regulated type I interferon production, which is a common mechanism by which viruses are able to establish chronic infections. They also observed that ORF1 initially increased production of inflammatory cytokines after infection, while another viral protein, ORF2, did not. The ORF1 protein also appears to use mechanisms utilized by other viruses to suppress and evade the immune system. For example, SOCS-1 and PD-1 suppress T-cell responses, and thus are upregulated by both herpes simplex virus and the hepatitis C core protein in order to suppress the immune system and avoid clearance. Figure 3 shows that ORF1 up-regulated both of these proteins six hours post infection, shown by the fold change of each of the proteins, suggesting that anelloviruses may evade the immune system using a similar mechanism [[#References|[16]]]. <br> | ||
[[Image:SOCS.jpg|thumb|300px|right|Figure 3. Treatment with ORF1 increased expression of SOCS-1 and PD-1 six hours post infection [https://www.sciencedirect.com/science/article/pii/S0168170216300892?via%3Dihub (Singh and Ramamoorthy 2016)] [[#References|[16]]]. SOCS-1 and PD-1 suppress T-cell responses, suggesting this could account for how anelloviruses correlate with immunosuppression and evade immune clearance.]] | |||
<br> | <br> ORF2, another viral protein encoded by anelloviruses, has been shown to suppress NFκB, which is necessary for expression of inflammatory genes such as IL-6 and IL-8. Additionally, replication intermediates of anelloviruses can stimulate the immune system. Specifically, heterodimers of guanosine and cytosine, which are not present in mammalian DNA, can be detected by toll-like receptor 9 and either stimulate or inhibit production of inflammatory cytokines [[#References|[9]]]. <br> | ||
<br> | <br> Due the ability of anelloviruses to interact with the immune system and cytokine signaling, some have suggested that they may play a role in asthma development. While higher anellovirus loads have been found to correlate with acute respiratory diseases, it is still uncertain whether these viruses play a role. However, in children with respiratory diseases, the airways were found to be a site of replication for anelloviruses and higher viral loads correlated with more severe cases. Further research is necessary before the role of these viruses in inflammatory diseases is clear [[#References|[9]]]. <br> | ||
==Correlation with transplant outcomes== | ==Correlation with transplant outcomes== | ||
<br>Recent research suggests that anellovirus loads could be used as a predictor of transplant outcomes. High levels of anellovirus are associated with immune suppression, while low levels are correlated with immunocompetence. It had previously been found that lung transplant patients had increased anellovirus levels in their bronchoalveolar fluid and in their serum | <br>Recent research suggests that anellovirus loads could be used as a predictor of transplant outcomes. High levels of anellovirus are associated with immune suppression, while low levels are correlated with immunocompetence. It had previously been found that lung transplant patients had increased anellovirus levels in their bronchoalveolar fluid and in their serum after transplant [[#References|[20]]]. Additionally, low plasma anellovirus loads have been found to be associated with acute rejection in heart transplant recipients. This makes anelloviruses a potential marker of chronic rejection, as low plasma anellovirus levels may suggest later rejection.<br> | ||
[[Image:AnelloACR.png|thumb|300px|right|Correlation between relative anellovirus load and acute cellular rejection (ACR) after pediatric lung transplant. Those with higher anellovirus loads than average had a lower chance of rejecting the graft for all time points after transplantation ( | [[Image:AnelloACR.png|thumb|300px|right|Figure 4. Correlation between relative anellovirus load and acute cellular rejection (ACR) after pediatric lung transplant. Those with higher anellovirus loads than average had a lower chance of rejecting the graft for all time points after transplantation [https://onlinelibrary.wiley.com/doi/epdf/10.1111/petr.13069 (Blatter et al. 2018)] [[#References|[3]]].]] | ||
<br> Blatter et al. investigated the impact of both alphatorqueviruses and betatorqueviruses on the outcome of lung transplants in a pediatric cohort of 57 children. They hypothesized that high levels of anellovirus in the blood would be associated with lower levels of rejection and an overall better outcome. Additionally, they predicted that different genera of anellovirus might be associated with different outcomes, as previous research found that alphatorqueviruses correlated with fever in children but betatorqueviruses did not. <br> | <br> Blatter et al. investigated the impact of both alphatorqueviruses and betatorqueviruses on the outcome of lung transplants in a pediatric cohort of 57 children over 18 months post transplant. They hypothesized that high levels of anellovirus in the blood would be associated with lower levels of rejection and an overall better outcome. Additionally, they predicted that different genera of anellovirus might be associated with different outcomes, as previous research found that alphatorqueviruses correlated with fever in children but betatorqueviruses did not [[#References|[3]]]. <br> | ||
[[Image:Graftrejection.png|thumb|300px|right|Correlation between anellovirus load and graft rejection. De Vlaminck et al. found that at all time points, non-rejecting patients had higher anellovirus loads than rejecting patients (2013).]] | [[Image:Graftrejection.png|thumb|300px|right|Figure 5. Correlation between anellovirus load and graft rejection. De Vlaminck et al. found that at all time points, non-rejecting patients had higher anellovirus loads than rejecting patients [https://www.cell.com/cell/fulltext/S0092-8674(13)01352-4 (De Vlaminck et al. 2013)] [[#References|[5]]].]] | ||
<br> Blatter et al. found that alphatorquevirus levels were higher than betatorquevirus levels in all of the patients, but the load of each increased over the course of the study, | <br> Blatter et al. found that alphatorquevirus levels were higher than betatorquevirus levels in all of the patients, but the load of each increased over the course of the 18-month study. As shown in figure 4, those with high anellovirus loads relative to the control consistently had a higher chance of survival without acute cellular rejection than those with lower anellovirus loads at all time points during the study. The blue line represents those with lower relative viral loads, and their chance of survival without rejection decreases more drastically over time than those with higher relative loads, shown in red. They found that low alphatorquevirus loads were associated with acute rejection, while low betatorquevirus levels were correlated with negative long term outcomes, such as death or re-transplant. Similar results were found previously by De Vlaminck et al., who observed that from 1 to 16.5 months post-transplant, patients with rejecting outcomes consistently had a lower anellovirus load than non-rejecting patients [[#References|[5]]]. Figure 5 shows that those who did not reject grafts, represented in blue, consistently had higher anellovirus loads than those who did reject the graft, shown in red. The inset image of the figure shows that as incidence of infection went up, incidence of rejection went down, which the authors use to argue that anellovirus loads correlate with immunosuppression. Additionally, Abbas et al. recently found that anellovirus levels were 100-fold higher in donor lungs [[#References|[1]]]. This could be due to the corticosteroids given to organ donors to modulate inflammation after brain death, which suppress the immune system and allow for anellovirus levels to rise. Alternatively, donor lungs come from critically ill patients that may already have weakened immune systems, which could also explain the high levels of anelloviruses. Abbas et al. also observed that while absolute anellovirus levels did not significantly differ between patients that rejected or did not rejected transplant, those who ultimately had primary graft dysfunction had a smaller increase in anellovirus levels after transplant. The authors hypothesized that because primary graft dysfunction involves immune activation, this might limit an increase in anelloviruses, as the immune system would be active and able to repress the viruses. <br> | ||
<br> | <br> Blatter et al. also found distinct phylogenetic clusters of anelloviruses in patients pre-transplant and in controls when compared to post-transplant patients. It is still unclear whether anelloviruses directly interact with the immune system, but this study suggests that anellovirus load can still be a useful biomarker for prediction of transplant outcomes. Additionally, as discussed above, there is evidence that anelloviruses may be able to affect the immune system via miRNAs. <br> | ||
<br> In patients receiving high dose chemotherapy coupled with autologous stem cell transplantation, an increase in anellovirus load is observed post treatment. Additionally, this increase correlated with an increase in CD8+ T cells, which can help fight cancer. | <br> In patients receiving high dose chemotherapy coupled with autologous stem cell transplantation, an increase in anellovirus load is observed post treatment. Additionally, this increase correlated with an increase in CD8+ T cells, which can help fight cancer. Anellovirus loads returned to baseline around 100 days post transplant, and the authors used this to hypothesize about when patients have returned to immunocompetent status. Anellovirus loads offer an opportunity for clinicians to make informed decisions about when their patients will have the best outcomes for transplants [[#References|[7]]]. Interestingly, there has been no observed effect of chemotherapy on anellovirus loads thus far. Some hypothesize that high anellovirus loads may increase the risk of graft-versus-host disease for stem cell transplant patients, as the virus could drive inflammatory cytokine production [[#References|[13]]]. However, another study measured anellovirus levels in those receiving allogeneic hematopoietic stem cell transplantation and found that anellovirus loads did not predict patient risk for complications [[#References|[19]]]. <br> | ||
==Correlation with AIDS progression== | |||
<br>Anellovirus loads likely increase post-transplant due to the immune suppression that is essential for successful transplant. Thus, while high anellovirus loads are correlated with better transplant outcomes because it means that the immune system is weakened and likely won't reject the graft, in general high anellovirus loads are correlated with disease. For example, it has also been found to correlate with the progression of AIDS in HIV-infected patients. Thom and Petrik found that patients with AIDS consistently had higher anellovirus loads than HIV positive and negative patients [[#References|[18]]]. They also found an inverse relationship between the levels of CD4 T-cells and anellovirus loads. CD4 T-cells, also known as T helper cells, help activate cytotoxic effector T-cells, which are part of the adaptive immune system that function to destroy pathogens. Thus, the correlation between higher anellovirus loads and lower CD4 T-cells suggests that higher viral loads promote or correlate with immunosuppression. While this can be a useful marker for transplant patients, high viral loads are still a marker of a suppressed immune system. <br> | |||
==Conclusion== | ==Conclusion== | ||
<br> | <br>Anelloviruses are ancient and persistent viruses, suggesting that they have been a part of human and animal populations for a great deal of our evolution. Whether or not these viruses are pathogenic has yet to be discerned, but to date there is little evidence for direct pathogenesis. This raises the question of what role these viruses play within animal systems. Anelloviruses have been observed to interact with aspects of the immune system, suggesting that they may have co-evolved to play a role in immune modulation. Phylogenetic analysis has suggested that anelloviruses originated in non-primates, but it is still unknown whether they are a result of co-evolution with their variety of hosts over time or if they emerged more recently and have diversified rapidly [[#References|[4]]]. Anelloviruses that infect humans and those that infect mammals have similar genomes and transcriptional organization, lending support to the co-evolution hypothesis, but anelloviruses from different species still have very little sequence homology [[#References|[12]]]. Thus, anelloviruses from humans and animals appear divergent in phylogenetic analysis. Additionally, the high diversity of these viruses makes it difficult to hypothesize about their origins. <br> | ||
<br> Because no direct link to disease has been found, some have proposed that anelloviruses are part of the human microbiota, suggesting that they have a commensal or possibly even mutualistic relationship with their hosts [[#References|[12]]]. The presence of anelloviruses in feces suggests that they may even play a role in the microbiome within the human gut, where they have also been found using metagenomics [[#References|[2]]]. However, the diverse population of microbes and viruses within the human gastrointestinal tract makes it hard to discern the role of each microbe within that microenvironment. <br> | |||
<br> While anellovirus loads have shown promise as a marker of immune status, there are many obstacles before they can be used diagnostically. The difficulty of growing these viruses in culture has prevented them from being studied in as much depth, and their complex interactions with the host make it difficult to discern their functions. Detection of anellovirus infection is relatively easy using quantitative real-time PCR and primers designed from the untranslated region of the viral genome, which is highly conserved. However, because these viruses are so diverse and heterogeneous, it is possible that not all anellovirus species have the same effect on the host, and thus may need to be differentiated before being used as immune diagnostics [[#References|[8]]]. <br> | |||
==References== | ==References== |
Latest revision as of 13:55, 11 May 2018
Introduction
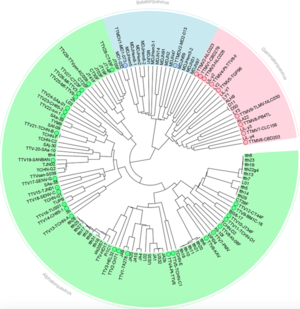
By Julia Josowitz
What are anelloviruses and what is our current understanding of their interactions with humans?
Anelloviruses are small, circular viruses found in blood plasma. They are single stranded and negative-sense DNA viruses, which means that its DNA can be directly transcribed into mRNA, as its DNA is the "template" strand. While they are not known to cause any harm, high viral loads are associated with immune suppression and diseases that correlate with immune suppression, such as hepatitis, cancer, and autoimmune diseases [3]. The first anellovirus discovered was torque teno virus (TTV) in 1997, which was initially believed to be part of the pathology of hepatitis, but since then these viruses have been found to be relatively widespread and heterogeneous, with no known pathogenicity [14][17]. TTV is still used as the prototypical anellovirus for many studies today, and it is one of the most prevalent. Since their discovery, it has become clear that these viruses are very ubiquitous, representing about 70% of the viruses found within human blood and tissues [9].
Within the family Anelloviridae there are twelve known genera, but originally there were thought to be three prototypical genera: Alphatorquevirus, Betatorquevirus, and Gammatorquevirus. Alphatorquevirus includes TTV, while Betatorquevirus includes torque teno mini virus (TTMV) and Gammatorquevirus includes torque teno midi virus (TTMDV). The high mutation rates and diversity have led to high-throughput sequencing categorizing the virus into even more genera [4]. These viruses have also been found in cats, dogs, and even pigs and bats. In humans, these viruses are very prevalent, with more than 80% of the population infected, and they appear to establish persistent infections that remain within the body for the rest of the human life. As shown in Figure 1, they are also very diverse, with at least 41 different species infecting humans as of 2012 [6]. Despite this diversity, TTV-8 is currently thought to be the most prevalent anellovirus in humans [8]. Although these viruses remain in the body after infection and infect such a large part of the population, their mechanisms of evading the host immune system are unknown. However, their ability to avoid immune clearance and their prevalence suggest an almost commensal relationship with the host.
Anelloviruses are unenveloped viruses that have a capsid made of 12 pentameric capsomers. With microscopy, it appears to be a spherical particle and it has icosahedral symmetry typical of many viruses [12]. There is no evidence of a lipid envelope, which usually includes proteins that the virus uses to bind host cells. Additionally, nonenveloped viruses tend to be more stable, which likely contributes to the persistence of these viruses within the body. Virion particle sizes vary between genera, with a range from 30 to 50 nm for TTV, but less than 30 nm for TTMV. Anelloviruses are currently unable to be grown in vitro due to lack of compatible cells systems, so the crystal structure of TTV has not been solved and much is unknown about the life cycle of these viruses. It is believed that they replicate within the bone marrow and liver of humans because double stranded replicative intermediates have been found in these tissues, indicating that the single-stranded virus is using the host machinery to replicate there. Anelloviruses have also been found to reside within peripheral blood mononuclear cells, and recent studies have suggested that under certain conditions, the virus may also be able to replicate within these cells, as double stranded intermediates have been found [18].
Transmission and correlation with disease
While the main transmission route is believed to be blood, there is evidence of multiple other routes. For example, anelloviruses are present in pharyngeal mucus, which suggests that they can also be disseminated via coughing or sneezing. This could help explain how they are so prevalent, as the virus could spread via droplets. Anelloviruses have also been found in the fecal matter of infected organisms and have been detected in sewage water, in addition to some meat products, which suggests an oral route of transmission. These viruses have been found in high numbers in the cervical epithelium, suggesting that they might be transmitted through sexual contact. It is possible that viruses are transmitted from mother to offspring, as the viruses have been found in amniotic fluid and umbilical cord blood, and the newborn viral genotype usually resembles the mother. This could also help explain the prevalence of the viruses in populations, as the virus would consistently be transferred from mother to child. However, it is also possible that newborns become infected early in life simply through contact with the mother [17]. Finally, because these viruses are prevalent in both animals and humans, it has been suggested that new genera of anelloviruses could arise due to anthropozoonosis, the transmission of pathogens from humans to animals or vice versa, introducing another source of transmission [4].
In addition to the prevalence of these viruses in the human population, they have been found to correlate with many diseases, such as hepatitis, HIV, cancer, asthma, and other autoimmune disorders. Patients with both hepatitis and TTV have higher mortality rates than those with hepatitis alone, but to date there is no evidence that the anellovirus contributes to the disease progression. Despite the higher mortality rates, there is no histopathological evidence that co-infection with TTV affects the liver in a different way than hepatitis alone. While TTV has also been detected in neoplastic tissues, it is unclear whether it contributes to cancer progression. Recent research has suggested that the small anellovirus DNA fragments found in human serum could be pathogenic, like geminiviruses in plants, but comprehensive studies of the interactions between the viral DNA and the hosts have yet to be conducted [11]. Due to the prevalence of these viruses, it is difficult to determine whether they are truly pathogenic and more research must be done to determine their role.
Genome
The anellovirus genome is made of a circular single stranded DNA molecule that has a GC rich region of 117 nucleotides. Genome size varies based on the host, but the TTV genome ranges from 3.6 to 3.9 kilobases [12]. Of this, there is a 1.2 kb untranslated region and a 2.6 kb coding region. The untranslated region has been highly conserved, suggesting that it likely plays a role in viral replication. Interestingly, the coding region has two large open reading frames, the first of which encodes a 770 amino acid residue protein and another that encodes a slightly smaller peptide in TTV. These reading frames vary between viral samples. The viral genome produces three viral proteins, shown in Figure 2; the largest viral protein, ORF1, likely encodes the capsid, but the function of the other two is unknown [16]. Anellovirus is hypothesized to replicate using the rolling-circle method.
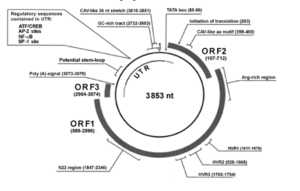
The genome of anellovirus is very heterogeneous, with high divergence between viral samples. Of the human TTVs in the genus Alphatorquevirus, there were at least 29 known species as of 2015, with more than 40 different genotypes. More specifically, ORF1, which encodes a viral protein, has very high variability with three regions that often experience insertions or deletions, increasing the variability between viruses [12]. Different genotypes can infect the same host at the same time, even within the same tissue. Researchers hypothesize that this may be due to a high mutation rate in TTV. This is uncommon for DNA viruses, which use the host’s own replicative machinery, making them less error-prone and the chance of mistakes more unlikely. However, research in single stranded DNA viruses such as parvoviruses has found that the single stranded nature of the genome and its ability to encode replication proteins may account for high rates of mutation, as its own replication machinery could be more error-prone. Thus, this could explain the high rates of mutation in anelloviruses.
Recently, it has also been proposed that intra-genomic recombination could account for the heterogeneity of the Anellovirus genome. It has been suggested that TTV has co-evolved with the host for over millions of years, allowing for great variability. However, this same variability makes phylogenetic analysis difficult, as will be discussed later.
Finally, the transmission of these viruses between humans and animals could also contribute to the high variability.
Potential interactions with the immune system
Other DNA viruses are known to stimulate immune cells to produce inflammatory cytokines, so Rocchi et al. studied whether TTVs could also have a direct effect on immune system signaling [15]. More specifically, they tested whether TTV DNA alone could have effects on the immune system. They used lipofectin, which incorporates DNA and protects it from nucleases, to simulate a viral capsid. Spleen cells were treated with lipofectin coupled with TTV DNA, which resulted in an increase in inflammatory cytokines. These results suggest that TTV DNA can directly effect transcription of inflammatory genes within target cells. Inhibitors were used to determine through which receptor TTV DNA was able to change host transcription. Toll-like receptor 9 was found to be the main mediator of the TTV DNA interaction. Toll-like receptor 9 is expressed on phagocytic cells, such as macrophages, as well as antigen-presenting cells, and it binds bacterial and viral DNA. Binding of foreign DNA triggers production of cytokines by the immune cell; thus, through toll-like receptor 9, these results suggest that TTV DNA can directly affect the immune system.
Anelloviruses have also been suggested to modulate the host immune system using micro RNAs (miRNAs). miRNAs are small noncoding RNAs (around 22 nucleotides) that play a role in posttranscriptional gene regulation. Viruses from the herpesvirus and retrovirus families have been found to encode viral miRNAs, and these viruses are also characterized by the ability to establish a persistent infection in their host, much like anelloviruses. It has also been suggested that viral miRNAs may play a role in immune evasion. Kincaid et al. developed computational methods to predict the possibility of miRNAs from the primary sequence of genes [10]. Their modeling predicted multiple miRNAs encoded by TTVs, and by engineering recombinant TTVs capable of expressing an RNA transgene in addition to the miRNA, they were able to identify a potential target of the viral miRNA, the gene N-myc interactor (NMI). The NMI gene modulates immune signaling, such as cytokines and interferon, which also stimulate the gene through feedback, making it a key regulator of the body's inflammatory response that eliminates pathogens. The authors suggest that the TTV miRNA helps the virus manipulate the immune system by targeting NMI to inhibit interferon and promote immune evasion, as the signaling molecules are suppressed and no immune response is activated. This could help explain how these viruses are so persistent in the human body and avoid immune clearance, as they are able to produce miRNAs that inhibit inflammatory responses of the immune system, which allow them to avoid elimination. However, while all of the human TTVs were found to encode possible miRNAs, none were observed in TTMDVs or TTMVs.
Research has also sought to identify the role of the proteins encoded by the viral genome. In a study of swine anelloviruses, it was found that the large viral protein produced by anelloviruses, termed ORF1, down-regulated type I interferon production, which is a common mechanism by which viruses are able to establish chronic infections. They also observed that ORF1 initially increased production of inflammatory cytokines after infection, while another viral protein, ORF2, did not. The ORF1 protein also appears to use mechanisms utilized by other viruses to suppress and evade the immune system. For example, SOCS-1 and PD-1 suppress T-cell responses, and thus are upregulated by both herpes simplex virus and the hepatitis C core protein in order to suppress the immune system and avoid clearance. Figure 3 shows that ORF1 up-regulated both of these proteins six hours post infection, shown by the fold change of each of the proteins, suggesting that anelloviruses may evade the immune system using a similar mechanism [16].
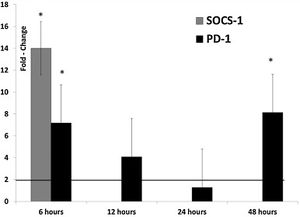
ORF2, another viral protein encoded by anelloviruses, has been shown to suppress NFκB, which is necessary for expression of inflammatory genes such as IL-6 and IL-8. Additionally, replication intermediates of anelloviruses can stimulate the immune system. Specifically, heterodimers of guanosine and cytosine, which are not present in mammalian DNA, can be detected by toll-like receptor 9 and either stimulate or inhibit production of inflammatory cytokines [9].
Due the ability of anelloviruses to interact with the immune system and cytokine signaling, some have suggested that they may play a role in asthma development. While higher anellovirus loads have been found to correlate with acute respiratory diseases, it is still uncertain whether these viruses play a role. However, in children with respiratory diseases, the airways were found to be a site of replication for anelloviruses and higher viral loads correlated with more severe cases. Further research is necessary before the role of these viruses in inflammatory diseases is clear [9].
Correlation with transplant outcomes
Recent research suggests that anellovirus loads could be used as a predictor of transplant outcomes. High levels of anellovirus are associated with immune suppression, while low levels are correlated with immunocompetence. It had previously been found that lung transplant patients had increased anellovirus levels in their bronchoalveolar fluid and in their serum after transplant [20]. Additionally, low plasma anellovirus loads have been found to be associated with acute rejection in heart transplant recipients. This makes anelloviruses a potential marker of chronic rejection, as low plasma anellovirus levels may suggest later rejection.

Blatter et al. investigated the impact of both alphatorqueviruses and betatorqueviruses on the outcome of lung transplants in a pediatric cohort of 57 children over 18 months post transplant. They hypothesized that high levels of anellovirus in the blood would be associated with lower levels of rejection and an overall better outcome. Additionally, they predicted that different genera of anellovirus might be associated with different outcomes, as previous research found that alphatorqueviruses correlated with fever in children but betatorqueviruses did not [3].
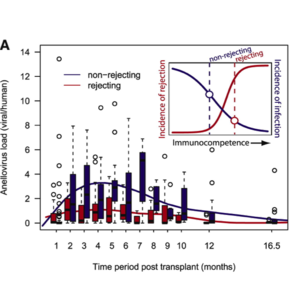
Blatter et al. found that alphatorquevirus levels were higher than betatorquevirus levels in all of the patients, but the load of each increased over the course of the 18-month study. As shown in figure 4, those with high anellovirus loads relative to the control consistently had a higher chance of survival without acute cellular rejection than those with lower anellovirus loads at all time points during the study. The blue line represents those with lower relative viral loads, and their chance of survival without rejection decreases more drastically over time than those with higher relative loads, shown in red. They found that low alphatorquevirus loads were associated with acute rejection, while low betatorquevirus levels were correlated with negative long term outcomes, such as death or re-transplant. Similar results were found previously by De Vlaminck et al., who observed that from 1 to 16.5 months post-transplant, patients with rejecting outcomes consistently had a lower anellovirus load than non-rejecting patients [5]. Figure 5 shows that those who did not reject grafts, represented in blue, consistently had higher anellovirus loads than those who did reject the graft, shown in red. The inset image of the figure shows that as incidence of infection went up, incidence of rejection went down, which the authors use to argue that anellovirus loads correlate with immunosuppression. Additionally, Abbas et al. recently found that anellovirus levels were 100-fold higher in donor lungs [1]. This could be due to the corticosteroids given to organ donors to modulate inflammation after brain death, which suppress the immune system and allow for anellovirus levels to rise. Alternatively, donor lungs come from critically ill patients that may already have weakened immune systems, which could also explain the high levels of anelloviruses. Abbas et al. also observed that while absolute anellovirus levels did not significantly differ between patients that rejected or did not rejected transplant, those who ultimately had primary graft dysfunction had a smaller increase in anellovirus levels after transplant. The authors hypothesized that because primary graft dysfunction involves immune activation, this might limit an increase in anelloviruses, as the immune system would be active and able to repress the viruses.
Blatter et al. also found distinct phylogenetic clusters of anelloviruses in patients pre-transplant and in controls when compared to post-transplant patients. It is still unclear whether anelloviruses directly interact with the immune system, but this study suggests that anellovirus load can still be a useful biomarker for prediction of transplant outcomes. Additionally, as discussed above, there is evidence that anelloviruses may be able to affect the immune system via miRNAs.
In patients receiving high dose chemotherapy coupled with autologous stem cell transplantation, an increase in anellovirus load is observed post treatment. Additionally, this increase correlated with an increase in CD8+ T cells, which can help fight cancer. Anellovirus loads returned to baseline around 100 days post transplant, and the authors used this to hypothesize about when patients have returned to immunocompetent status. Anellovirus loads offer an opportunity for clinicians to make informed decisions about when their patients will have the best outcomes for transplants [7]. Interestingly, there has been no observed effect of chemotherapy on anellovirus loads thus far. Some hypothesize that high anellovirus loads may increase the risk of graft-versus-host disease for stem cell transplant patients, as the virus could drive inflammatory cytokine production [13]. However, another study measured anellovirus levels in those receiving allogeneic hematopoietic stem cell transplantation and found that anellovirus loads did not predict patient risk for complications [19].
Correlation with AIDS progression
Anellovirus loads likely increase post-transplant due to the immune suppression that is essential for successful transplant. Thus, while high anellovirus loads are correlated with better transplant outcomes because it means that the immune system is weakened and likely won't reject the graft, in general high anellovirus loads are correlated with disease. For example, it has also been found to correlate with the progression of AIDS in HIV-infected patients. Thom and Petrik found that patients with AIDS consistently had higher anellovirus loads than HIV positive and negative patients [18]. They also found an inverse relationship between the levels of CD4 T-cells and anellovirus loads. CD4 T-cells, also known as T helper cells, help activate cytotoxic effector T-cells, which are part of the adaptive immune system that function to destroy pathogens. Thus, the correlation between higher anellovirus loads and lower CD4 T-cells suggests that higher viral loads promote or correlate with immunosuppression. While this can be a useful marker for transplant patients, high viral loads are still a marker of a suppressed immune system.
Conclusion
Anelloviruses are ancient and persistent viruses, suggesting that they have been a part of human and animal populations for a great deal of our evolution. Whether or not these viruses are pathogenic has yet to be discerned, but to date there is little evidence for direct pathogenesis. This raises the question of what role these viruses play within animal systems. Anelloviruses have been observed to interact with aspects of the immune system, suggesting that they may have co-evolved to play a role in immune modulation. Phylogenetic analysis has suggested that anelloviruses originated in non-primates, but it is still unknown whether they are a result of co-evolution with their variety of hosts over time or if they emerged more recently and have diversified rapidly [4]. Anelloviruses that infect humans and those that infect mammals have similar genomes and transcriptional organization, lending support to the co-evolution hypothesis, but anelloviruses from different species still have very little sequence homology [12]. Thus, anelloviruses from humans and animals appear divergent in phylogenetic analysis. Additionally, the high diversity of these viruses makes it difficult to hypothesize about their origins.
Because no direct link to disease has been found, some have proposed that anelloviruses are part of the human microbiota, suggesting that they have a commensal or possibly even mutualistic relationship with their hosts [12]. The presence of anelloviruses in feces suggests that they may even play a role in the microbiome within the human gut, where they have also been found using metagenomics [2]. However, the diverse population of microbes and viruses within the human gastrointestinal tract makes it hard to discern the role of each microbe within that microenvironment.
While anellovirus loads have shown promise as a marker of immune status, there are many obstacles before they can be used diagnostically. The difficulty of growing these viruses in culture has prevented them from being studied in as much depth, and their complex interactions with the host make it difficult to discern their functions. Detection of anellovirus infection is relatively easy using quantitative real-time PCR and primers designed from the untranslated region of the viral genome, which is highly conserved. However, because these viruses are so diverse and heterogeneous, it is possible that not all anellovirus species have the same effect on the host, and thus may need to be differentiated before being used as immune diagnostics [8].
References
Edited by Julia Josowitz of Joan Slonczewski for BIOL 238 Microbiology, 2018, Kenyon College.