Methanosarcina barkeri CM1 and its environmental impacts: Difference between revisions
Slonczewski (talk | contribs) No edit summary |
|||
(102 intermediate revisions by 3 users not shown) | |||
Line 1: | Line 1: | ||
==Introduction== | ==Introduction== | ||
<i>Methanosarcina barkeri</i> CM1 is a species of methanogenic archaea of the CM1 strain, and like many other methanogens, is characterized by its production of methane as it generates energy. The <i>Methanosarcina barkeri</i> species has a coccoid shape and is nonmotile (Figure 1).<ref> | <i>Methanosarcina barkeri</i> CM1 is a species of methanogenic archaea of the CM1 strain, and like many other methanogens, is characterized by its production of methane as it generates energy. The <i>Methanosarcina barkeri</i> species has a coccoid shape and is nonmotile (Figure 1).<ref name = (1)>Hook, Sarah E et al. “Methanogens: methane producers of the rumen and mitigation strategies.” Archaea (Vancouver, B.C.) vol. 2010 945785. 30 Dec. 2010, doi:10.1155/2010/945785. https://www.ncbi.nlm.nih.gov/pmc/articles/PMC3021854/</ref> The archaea was found within the rumen of a Friesian cow on a diet of clover and ryegrass in New Zealand and since then its genome has led to discoveries in rumen methanogen diversity as well as environmental problems with the production of methane.<ref>Jarvis, G., Strömpl, C., Burgess, D. et al. Isolation and Identification of Ruminal Methanogens from Grazing Cattle. Curr Microbiol 40, 327–332 (2000).[https://link.springer.com/article/10.1007/s002849910065#citeas]</ref> | ||
Ruminant animals, such as this cow, through evolution have developed a digestive system that utilizes microbes to ferment plant fiber (2), as well as to produce digestible nutrients necessary for the ruminant host animal. (3) The fermentation of fibers, sugars, and starches produces carbon dioxides, methane, and volatile fatty acids. (3) | [[Image:Morphotype.jpg|thumb|300px|right|<b>Figure 1:</b> Micrograph of <i>Methanosarcina barkeri</i> CM1 cells. This image was produced using Olympus Fluoview 10-ASW software. (In courtesy of Standards of Genomic Sciences) Link: https://pubmed.ncbi.nlm.nih.gov/26413197/#&gid=article-figures&pid=fig-3-uid-2]] | ||
Ruminant animals, such as this cow, through evolution have developed a digestive system that utilizes microbes to ferment plant fiber <ref name = (2)>Lambie, S.C., Kelly, W.J., Leahy, S.C. et al. The complete genome sequence of the rumen methanogen Methanosarcina barkeri CM1. Stand in Genomic Sci 10, 57 (2015). [https://link.springer.com/article/10.1186/s40793-015-0038-5#citeas]</ref>, as well as to produce digestible nutrients necessary for the ruminant host animal.<ref name = (3)>Owens F.N., Basalan M. (2016) Ruminal Fermentation. In: Millen D., De Beni Arrigoni M., Lauritano Pacheco R. (eds) Rumenology. Springer, Cham. https://doi.org/10.1007/978-3-319-30533-2_3 [https://link.springer.com/chapter/10.1007%2F978-3-319-30533-2_3]</ref> The fermentation of fibers, sugars, and starches produces carbon dioxides, methane, and volatile fatty acids (including acetate).<ref name = (3)/> Hydrogenotrophic methanogenesis generates methane from hydrogen gas and carbon dioxide. Acetoclastic methanogenesis produces methane from acetate. Acetoclastic methanogens are the main kind of methanogens in ruminants.<ref name = (2)/> | |||
==Genetics== | ==Genetics== | ||
<i>Methanosarcina barkeri</i> CM1 is a part of the domain Archaea, the phylum Euryarchaeota, the class Methanococci, the order Methanosarcinales, the family Methanosarcinaceae, the genus <i>Methanosarcina</i>, the species <i>Methanosarcina barkeri</i>, and the strain CM1. ( | <i>Methanosarcina barkeri</i> CM1 is a part of the domain Archaea, the phylum Euryarchaeota, the class Methanococci, the order Methanosarcinales, the family Methanosarcinaceae, the genus <i>Methanosarcina</i>, the species <i>Methanosarcina barkeri</i>, and the strain CM1.<ref name = (2)/> The genome of <i>Methanosarcina barkeri</i> CM1 is made up of an individual circular chromosome composed of 4,501,171 base pairs, and an approximate 39% guanine plus cytosine content. Approximately 70% of the genome consists of protein-coding genes at 3,523, with 3,655 genes total. The genome also has three CRISPR gene clusters and three type I restriction/modification systems. <ref name = (2)/> Restriction/modification systems are found in bacteria as well as other prokaryotes, and aid in preventing infection from external DNA by acting as immune systems.<ref>Libretexts. “1.3: Bacterial Restriction/Modification System.” Biology LibreTexts, 14 July 2020.</ref> <i>Methanosarcina barkeri</i> CM1 has two origins of replication within conserved genes (MCM1_3593 and MCM1_001), that are 95 kb (or 95,000 bp) apart, and does not have prophage or plasmid sequences. <ref name = (2)/> The <i>Methanosarcina barkeri</i> genome is more conserved closer to the origin of replication than other <i>Methanosarcina</i> species. Infact, within this genus there are interspecies gene similarities up to 95%.<ref>Maeder, Dennis L., et al. “The Methanosarcina Barkeri Genome: Comparative Analysis with Methanosarcina Acetivorans and Methanosarcina Mazei Reveals Extensive Rearrangement within Methanosarcinal Genomes.” Journal of Bacteriology, American Society for Microbiology Journals, 15 Nov. 2006, jb.asm.org/content/188/22/7922.</ref> Out of the known species of the genus <i>Methanosarcina</i>, <i>Methanosarcina barkeri</i> CM1 is related most closely to <i>Methanosarcina barkeri</i> Fusaro, another strain of the <i>Methanosarcina barkeri</i> species (Figure 2). <ref name = (2)/> The CM1 strain was isolated from bovine rumen and the Fusaro was isolated from freshwater sediment, with a difference in genome size of about 0.37 Mb (the Fusaro being the larger strain).<ref name = (2)/> | ||
[[Image:CM1 Tree.jpg|thumb|300px|left|<b>Figure 2:</b> Phylogenetic tree of multiple <i>Methanosarcina</i> species including the CM1 and Fusaro strains, each with the appropriate accession number. The Neighbor-Joining method determined the likely evolutionary history and the Kimura 2-parameter method determined the likely evolutionary distances. (In courtesy of Standards of Genomic Sciences).]] | [[Image:CM1 Tree.jpg|thumb|300px|left|<b>Figure 2:</b> Phylogenetic tree of multiple <i>Methanosarcina</i> species including the CM1 and Fusaro strains, each with the appropriate accession number. The Neighbor-Joining method determined the likely evolutionary history and the Kimura 2-parameter method determined the likely evolutionary distances. (In courtesy of Standards of Genomic Sciences) Link: https://pubmed.ncbi.nlm.nih.gov/26413197/#&gid=article-figures&pid=fig-2-uid-1 Neighbor-Joining method: https://academic.oup.com/mbe/article/4/4/406/1029664 Kimura 2-parameter method: https://pubmed.ncbi.nlm.nih.gov/7463489/.]] | ||
Unique to <i>Methanosarcina</i> species is the S-layer protein that surrounds the cytoplasm-containing membrane. (2) In a 2012 study conducted on S-layer and cell surface-layer proteins in methanogenic archaea, slmA1 genes for <i>Methanosarcina acetivorans</i>, <i>Methanosarcina barkeri</i>, and <i>Methanosarcina mazei</i> were both transcribed and translated at a high rate, indicating a similar control over expression of S-layer proteins. ( | Unique to <i>Methanosarcina</i> species is the S-layer protein that surrounds the cytoplasm-containing membrane. <ref name = (2)/> In a 2012 study conducted on S-layer and cell surface-layer proteins in methanogenic archaea, slmA1 genes for <i>Methanosarcina acetivorans</i>, <i>Methanosarcina barkeri</i>, and <i>Methanosarcina mazei</i> were both transcribed and translated at a high rate, indicating a similar control over expression of S-layer proteins.<ref name = (6)>Rohlin, Lars, et al. “Identification of the Major Expressed S-Layer and Cell Surface-Layer-Related Proteins in the Model Methanogenic Archaea: Methanosarcina Barkeri Fusaro and Methanosarcina Acetivorans C2A.” Archaea, Hindawi, 15 May 2012, www.hindawi.com/journals/archaea/2012/873589/.</ref> In addition, the Mbar_A1758 and MA0829 proteins were found in both <i>Methanosarcina barkeri</i> and <i>Methanosarcina acetivorans</i> to be the greatest expressed S-layer protein. <ref name = (6)/> Within the <i>Methanosarcina barkeri</i> species, the CM1 strain as well as the Fusaro have nine proteins that have the DUF1608 domain. <ref name = (2)/> | ||
Also in <i>Methanosarcina</i> genomes are groups of nitrogenase genes. <i>Methanosarcina barkeri</i> is able to fix nitrogen, with the CM1 strand containing two nif operons that make up nitrogenase cofactor biosynthesis genes and nitrogenase, including the MCM1_2924-2930 nitrogenase (molybdenum and iron-containing) and the MCM1_1063-1072 nitrogenase (vanadium and iron-containing). CM1 differs from both <i>Methanosarcina acetivorans</i> and <i>Methanosarcina barkeri</i> Fusaro in that its genes do not encode for the exclusively iron-containing nitrogenase. In addition, unlike <i>Methanosarcina barkeri</i>, there are no genes present in CM1 that enable production of gas vesicles. (2) | Also in <i>Methanosarcina</i> genomes are groups of nitrogenase genes. <i>Methanosarcina barkeri</i> is able to fix nitrogen, with the CM1 strand containing two nif operons that make up nitrogenase cofactor biosynthesis genes and nitrogenase, including the MCM1_2924-2930 nitrogenase (molybdenum and iron-containing) and the MCM1_1063-1072 nitrogenase (vanadium and iron-containing). CM1 differs from both <i>Methanosarcina acetivorans</i> and <i>Methanosarcina barkeri</i> Fusaro in that its genes do not encode for the exclusively iron-containing nitrogenase. In addition, unlike <i>Methanosarcina barkeri</i>, there are no genes present in CM1 that enable the production of gas vesicles. <ref name = (2)/> | ||
==Microbiome & Methanogenesis== | ==Microbiome & Methanogenesis== | ||
Common to both <i>Methanosarcina barkeri</i> CM1 and <i>Methanosarcina barkeri</i> Fusaro, as well as to other species of <i>Methanosarcina</i> and other methanogens, is the process of methanogenesis. Methanogenesis occurs in anaerobic environments, or without the presence of oxygen. ( | Common to both <i>Methanosarcina barkeri</i> CM1 and <i>Methanosarcina barkeri</i> Fusaro, as well as to other species of <i>Methanosarcina</i> and other methanogens, is the process of methanogenesis. Methanogenesis occurs in anaerobic environments, or without the presence of oxygen.<ref name = (7)>Feist, Adam M, Scholten, Johannes C M, Palsson, Bernhard Ø, Brockman, Fred J, Ideker, Trey, (2006) Modeling methanogenesis with a genome‐scale metabolic reconstruction of Methanosarcina barkeri. Molecular Systems Biology, 2. 2006.0004. doi: accession:10.1038/msb4100046[https://www.embopress.org/action/showCitFormats?doi=10.1038%2Fmsb4100046]</ref> Methanogens (a subset within archaea) produce energy by converting certain substrates, including methanol, hydrogen with CO<sub>2</sub>, or acetate, into methane.<ref name = (7)/> It has been found that <i>Methanosarcina barkeri</i> grows at the greatest rate and with the highest production on methanol. Within all of these pathways (hydrogenotrophic, acetoclastic, and methylotrophic), a methyl group is transferred to coenzyme M which is reduced to methane.<ref name = (2)/> The CM1 strand has the genes to utilize each method of methanogenesis (Figure 3).<ref>Gonnerman, M.C., Benedict, M.N., Feist, A.M., Metcalf, W.W. and Price, N.D. (2013), Genomically and biochemically accurate metabolic reconstruction of Methanosarcina barkeri Fusaro, iMG746. Biotechnology Journal, 8: 1070-1079.[https://onlinelibrary.wiley.com/action/showCitFormats?doi=10.1002%2Fbiot.201200266] </ref> | ||
[[Image:CM1 Methanogenesis.jpg|thumb|300px|right|<b>Figure 3:</b> Three methods of methanogenesis, all of which <i>Methanosarcina barkeri</i> CM1 use. (In courtesy of Standards of Genomic Sciences).]] | [[Image:CM1 Methanogenesis.jpg|thumb|300px|right|<b>Figure 3:</b> Three methods of methanogenesis, all of which <i>Methanosarcina barkeri</i> CM1 use. (In courtesy of Standards of Genomic Sciences) Link: https://pubmed.ncbi.nlm.nih.gov/26413197/#&gid=article-figures&pid=fig-3-uid-2]] | ||
Rumen microorganism methanogenesis has become increasingly relevant on a global scale as the raising of ruminants has become more and more commonplace. Methanogenesis occurs by methanogens residing in an animal’s rumen and hindgut. (1) Approximately 89% of ruminant-produced methane comes from the rumen and exits the animal’s body by way of the nose and mouth (Figure 4). (1) Raising animals such as cattle and sheep not only leads to a buildup of greenhouse gases, but also results in a loss of energy through the significant amount of food necessary to sustain ruminants.<ref | Rumen microorganism methanogenesis has become increasingly relevant on a global scale as the raising of ruminants has become more and more commonplace. Methanogenesis occurs by methanogens residing in an animal’s rumen and hindgut.<ref name = (1)/> Approximately 89% of ruminant-produced methane comes from the rumen and exits the animal’s body by way of the nose and mouth (Figure 4).<ref name = (1)/> Raising animals such as cattle and sheep not only leads to a buildup of greenhouse gases, but also results in a loss of energy through the significant amount of food necessary to sustain ruminants.<ref name = (1)/> | ||
[[Image:Cow methanogenesis.jpg|thumb|300px|left|<b>Figure 4:</b> Methane production by methanogens in cattle and specific sources of ruminant methane emissions. Also shown is a comparison of methane emission per gram of protein for various livestock animals.]] | [[Image:Cow methanogenesis.jpg|thumb|300px|left|<b>Figure 4:</b> Methane production by methanogens in cattle and specific sources of ruminant methane emissions. Also shown is a comparison of methane emission per gram of protein for various livestock animals. Link: http://benisonmedia.com/a-discovery-to-lower-methane-emissions-from-livestock/]] | ||
Some of the most harmful greenhouse gases include nitrous oxide, carbon dioxide, ozone, and methane. Methane, however, while second in greenhouse gas abundance to carbon dioxide, is twenty-five times more potent than CO<sub>2</sub>, has an atmospheric lifetime of twelve years, and can increase tropospheric ozone and stratospheric water vapor content. (1) World wide- agriculture makes up one-fifth of the anthropogenic greenhouse effect and generates half of the total anthropogenic methane emissions ( | Some of the most harmful greenhouse gases include nitrous oxide, carbon dioxide, ozone, and methane. Methane, however, while second in greenhouse gas abundance to carbon dioxide, is twenty-five times more potent than CO<sub>2</sub>, has an atmospheric lifetime of twelve years, and can increase tropospheric ozone and stratospheric water vapor content.<ref name = (1)/> World wide-agriculture makes up one-fifth of the anthropogenic greenhouse effect and generates half of the total anthropogenic methane emissions.<ref>Ellis, J. L., et al. “Prediction of Methane Production from Dairy and Beef Cattle.” Journal of Dairy Science, vol. 90, no. 7, 2007, pp. 3456–66. Crossref, doi:10.3168/jds.2006-675.[https://www.journalofdairyscience.org/article/S0022-0302(07)71795-2/fulltext#articleInformation]</ref> | ||
==Methane | ==Reduction of Methane Emissions== | ||
One method of methane emission mitigation is the use of vaccines against rumen methanogens. Vaccines either lessen the activity of rumen methanogens or decrease their numbers, and would be more cost effective and manageable in livestock that graze. The general idea of a vaccine would include a significant salivary antibody response to introduce antibodies to the rumen and stop some methanogen function. ( | One method of methane emission mitigation is the use of vaccines against rumen methanogens. Vaccines either lessen the activity of rumen methanogens or decrease their numbers, and would be more cost effective and manageable in livestock that graze. The general idea of a vaccine would include a significant salivary antibody response to introduce antibodies to the rumen and stop some methanogen function.<ref name = (10)>Buddle, Bryce M., et al. “Strategies to Reduce Methane Emissions from Farmed Ruminants Grazing on Pasture.” The Veterinary Journal, vol. 188, no. 1, 2011, pp. 11–17. Crossref, doi:10.1016/j.tvjl.2010.02.019.[https://www.sciencedirect.com/science/article/abs/pii/S1090023310000821?casa_token=w_C21FlR8vYAAAAA:7bzFiBjsKSt9oJhI84l1PsQUu5STHwx4EJZhxtJt3uKUHmceK6d0ZRIefpy0sJvq0_ekgnaw#b0385]</ref> In a study conducted on methane emissions from sheep with vaccinations specific to certain species and strains of rumen methanogens, vaccines caused a serum antibody response which altered methanogen diversity and population. The vaccine also completely or partially eliminated some strains of <i>Methanobrevibacter</i> (a methanogen genus).<ref>Williams, Yvette J., et al. “A Vaccine against Rumen Methanogens Can Alter the Composition of Archaeal Populations.” Applied and Environmental Microbiology, vol. 75, no. 7, 2009, pp. 1860–66. Crossref, doi:10.1128/aem.02453-08.[https://aem.asm.org/content/75/7/1860.full]</ref> | ||
Other methods of reducing methane emissions include the use of bacteriophages, homoacetogens, protozoa, and dry matter intake and nutrition. Bacteriophages’ ability to lyse host cells has proven an advantage helpful in curbing methanogenesis from specific methanogen species and strains. Bacteriophages, as is necessary for methanogen-reducing vaccines, specify particular host species or strains and when used to decrease methanogen diversity or populations, work against certain methanogens while leaving others undisturbed in the rumen. However, in order for an efficient use of phages against methanogens, many more phages must be sequenced in order to keep up with hosts, who evolve quickly in order to reduce their risk of infection. In addition, extreme phage specificity reduces the possibility of an effective methane decrease as many different methanogen species and strains exist in ruminants. ( | Other methods of reducing methane emissions include the use of bacteriophages, homoacetogens, protozoa, and dry matter intake and nutrition. Bacteriophages’ ability to lyse host cells has proven an advantage helpful in curbing methanogenesis from specific methanogen species and strains. Bacteriophages, as is necessary for methanogen-reducing vaccines, specify particular host species or strains and when used to decrease methanogen diversity or populations, work against certain methanogens while leaving others undisturbed in the rumen. However, in order for an efficient use of phages against methanogens, many more phages must be sequenced in order to keep up with hosts, who evolve quickly in order to reduce their risk of infection. In addition, extreme phage specificity reduces the possibility of an effective methane decrease as many different methanogen species and strains exist in ruminants.<ref name = (10)/> | ||
[[Image:Seaweed.jpg|thumb|300px|right|<b>Figure 5:</b> Photograph of aquaculture longline seaweed farming experiments in in Van Phong Bay, Vietnam.]] | [[Image:Seaweed.jpg|thumb|300px|right|<b>Figure 5:</b> Photograph of aquaculture longline seaweed farming experiments in in Van Phong Bay, Vietnam. Link: https://civileats.com/2019/06/03/can-we-grow-enough-seaweed-to-help-cows-fight-climate-change/]] | ||
Homoacetogens are bacteria that grow using H<sub>2</sub> as energy by reducing it to CO<sub>2</sub> and then acetate. In order to reduce methane loss from ruminant feed energy and increase animal efficiency, H<sub>2</sub> energy forms acetate and methane production would decrease. Normally, low amounts of H<sub>2</sub> in rumen results in fewer homoacetogens than methanogens. By increasing the concentration of homoacetogens in the rumen, more H<sub>2</sub> will be used by homoacetogens resulting in an overall methane reduction. Also related to H<sub>2</sub> use is protozoa, which transfers H<sub>2</sub> to methanogens. By removing or controlling rumen protozoa, less H<sub>2</sub> would be present for use in methanogenesis. Possible implementations of minimizing protozoa numbers include breeding entire herds free of protozoa (often done in isolation), the use of detergents to reduce protozoa (chemical defaunation), the use of oils, saponins, and tannins, and focus on diet. Diet changes could potentially cause changes that create an inhospitable environment for protozoa, such as a fluctuation in pH. Another possible diet change is an increased consumption of soluble sugars, which generally produce less methane than plant fibers. ( | Homoacetogens are bacteria that grow using H<sub>2</sub> as energy by reducing it to CO<sub>2</sub> and then acetate. In order to reduce methane loss from ruminant feed energy and increase animal efficiency, H<sub>2</sub> energy forms acetate and methane production would decrease. Normally, low amounts of H<sub>2</sub> in rumen results in fewer homoacetogens than methanogens. By increasing the concentration of homoacetogens in the rumen, more H<sub>2</sub> will be used by homoacetogens resulting in an overall methane reduction. Also related to H<sub>2</sub> use is protozoa, which transfers H<sub>2</sub> to methanogens. By removing or controlling rumen protozoa, less H<sub>2</sub> would be present for use in methanogenesis. Possible implementations of minimizing protozoa numbers include breeding entire herds free of protozoa (often done in isolation), the use of detergents to reduce protozoa (chemical defaunation), the use of oils, saponins, and tannins, and focus on diet. Diet changes could potentially cause changes that create an inhospitable environment for protozoa, such as a fluctuation in pH. Another possible diet change is an increased consumption of soluble sugars, which generally produce less methane than plant fibers.<ref name = (10)/> | ||
One challenge with changing ruminant diets is the massive production rates necessary for changes to influence overall methane emissions. A recent study examined the effect of seaweed in cattle feed on methane emissions from cows. Research dealt mostly with the Asparagopsis taxiformis species from Southern California | One challenge with changing ruminant diets is the massive production rates necessary for changes to influence overall methane emissions. A recent study examined the effect of seaweed in cattle feed on methane emissions from cows. Research dealt mostly with the <i>Asparagopsis taxiformis</i> species from Southern California and the possibility of growing the plant on a scale large enough to be incorporated into cattle feed. The challenge, however, is growing seaweed in aquaculture farms, as most seaweed farming occurs in only a few Asian nations (Figure 5).<ref>Wozniacka, Gosia. “Can We Grow Enough Seaweed to Help Cows Fight Climate Change?” Civil Eats, 19 Sept. 2019, civileats.com/2019/06/03/can-we-grow-enough-seaweed-to-help-cows-fight-climate-change.[https://civileats.com/2019/06/03/can-we-grow-enough-seaweed-to-help-cows-fight-climate-change/]</ref> | ||
==Conclusion== | ==Conclusion== | ||
<i>Methanosarcina barkeri</i> CM1 is one of many species of rumen methanogens. As the raising and consumption of ruminants increases, so too does the amount of food and water necessary to sustain this industry. | <i>Methanosarcina barkeri</i> CM1 is one of many species of rumen methanogens. As the raising and consumption of ruminants increases, so too does the amount of food and water necessary to sustain this industry. Additionally, because the CM1 strain, similar to other methanogens, can produce methane from multiple molecules, decreasing methane production from animals used for food throughout history and the world proves extremely challenging. However, as global warming becomes an increasing threat, efforts are ongoing to find new ways to keep this food source available while reducing greenhouse gas emissions. | ||
==References== | ==References== | ||
<references /> | <references /> | ||
<br>Edited by | <br>Edited by Emily Banthin, student of [mailto:slonczewski@kenyon.edu Joan Slonczewski] for [http://biology.kenyon.edu/courses/biol116/biol116_Fall_2013.html BIOL 116 Information in Living Systems], 2020, [http://www.kenyon.edu/index.xml Kenyon College]. | ||
<!--Do not edit or remove this line-->[[Category:Pages edited by students of Joan Slonczewski at Kenyon College]] | <!--Do not edit or remove this line-->[[Category:Pages edited by students of Joan Slonczewski at Kenyon College]] |
Latest revision as of 21:00, 22 December 2020
Introduction
Methanosarcina barkeri CM1 is a species of methanogenic archaea of the CM1 strain, and like many other methanogens, is characterized by its production of methane as it generates energy. The Methanosarcina barkeri species has a coccoid shape and is nonmotile (Figure 1).[1] The archaea was found within the rumen of a Friesian cow on a diet of clover and ryegrass in New Zealand and since then its genome has led to discoveries in rumen methanogen diversity as well as environmental problems with the production of methane.[2]

Ruminant animals, such as this cow, through evolution have developed a digestive system that utilizes microbes to ferment plant fiber [3], as well as to produce digestible nutrients necessary for the ruminant host animal.[4] The fermentation of fibers, sugars, and starches produces carbon dioxides, methane, and volatile fatty acids (including acetate).[4] Hydrogenotrophic methanogenesis generates methane from hydrogen gas and carbon dioxide. Acetoclastic methanogenesis produces methane from acetate. Acetoclastic methanogens are the main kind of methanogens in ruminants.[3]
Genetics
Methanosarcina barkeri CM1 is a part of the domain Archaea, the phylum Euryarchaeota, the class Methanococci, the order Methanosarcinales, the family Methanosarcinaceae, the genus Methanosarcina, the species Methanosarcina barkeri, and the strain CM1.[3] The genome of Methanosarcina barkeri CM1 is made up of an individual circular chromosome composed of 4,501,171 base pairs, and an approximate 39% guanine plus cytosine content. Approximately 70% of the genome consists of protein-coding genes at 3,523, with 3,655 genes total. The genome also has three CRISPR gene clusters and three type I restriction/modification systems. [3] Restriction/modification systems are found in bacteria as well as other prokaryotes, and aid in preventing infection from external DNA by acting as immune systems.[5] Methanosarcina barkeri CM1 has two origins of replication within conserved genes (MCM1_3593 and MCM1_001), that are 95 kb (or 95,000 bp) apart, and does not have prophage or plasmid sequences. [3] The Methanosarcina barkeri genome is more conserved closer to the origin of replication than other Methanosarcina species. Infact, within this genus there are interspecies gene similarities up to 95%.[6] Out of the known species of the genus Methanosarcina, Methanosarcina barkeri CM1 is related most closely to Methanosarcina barkeri Fusaro, another strain of the Methanosarcina barkeri species (Figure 2). [3] The CM1 strain was isolated from bovine rumen and the Fusaro was isolated from freshwater sediment, with a difference in genome size of about 0.37 Mb (the Fusaro being the larger strain).[3]
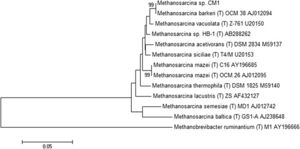
Unique to Methanosarcina species is the S-layer protein that surrounds the cytoplasm-containing membrane. [3] In a 2012 study conducted on S-layer and cell surface-layer proteins in methanogenic archaea, slmA1 genes for Methanosarcina acetivorans, Methanosarcina barkeri, and Methanosarcina mazei were both transcribed and translated at a high rate, indicating a similar control over expression of S-layer proteins.[7] In addition, the Mbar_A1758 and MA0829 proteins were found in both Methanosarcina barkeri and Methanosarcina acetivorans to be the greatest expressed S-layer protein. [7] Within the Methanosarcina barkeri species, the CM1 strain as well as the Fusaro have nine proteins that have the DUF1608 domain. [3]
Also in Methanosarcina genomes are groups of nitrogenase genes. Methanosarcina barkeri is able to fix nitrogen, with the CM1 strand containing two nif operons that make up nitrogenase cofactor biosynthesis genes and nitrogenase, including the MCM1_2924-2930 nitrogenase (molybdenum and iron-containing) and the MCM1_1063-1072 nitrogenase (vanadium and iron-containing). CM1 differs from both Methanosarcina acetivorans and Methanosarcina barkeri Fusaro in that its genes do not encode for the exclusively iron-containing nitrogenase. In addition, unlike Methanosarcina barkeri, there are no genes present in CM1 that enable the production of gas vesicles. [3]
Microbiome & Methanogenesis
Common to both Methanosarcina barkeri CM1 and Methanosarcina barkeri Fusaro, as well as to other species of Methanosarcina and other methanogens, is the process of methanogenesis. Methanogenesis occurs in anaerobic environments, or without the presence of oxygen.[8] Methanogens (a subset within archaea) produce energy by converting certain substrates, including methanol, hydrogen with CO2, or acetate, into methane.[8] It has been found that Methanosarcina barkeri grows at the greatest rate and with the highest production on methanol. Within all of these pathways (hydrogenotrophic, acetoclastic, and methylotrophic), a methyl group is transferred to coenzyme M which is reduced to methane.[3] The CM1 strand has the genes to utilize each method of methanogenesis (Figure 3).[9]
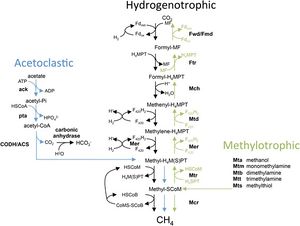
Rumen microorganism methanogenesis has become increasingly relevant on a global scale as the raising of ruminants has become more and more commonplace. Methanogenesis occurs by methanogens residing in an animal’s rumen and hindgut.[1] Approximately 89% of ruminant-produced methane comes from the rumen and exits the animal’s body by way of the nose and mouth (Figure 4).[1] Raising animals such as cattle and sheep not only leads to a buildup of greenhouse gases, but also results in a loss of energy through the significant amount of food necessary to sustain ruminants.[1]
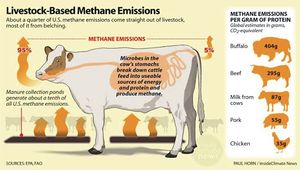
Some of the most harmful greenhouse gases include nitrous oxide, carbon dioxide, ozone, and methane. Methane, however, while second in greenhouse gas abundance to carbon dioxide, is twenty-five times more potent than CO2, has an atmospheric lifetime of twelve years, and can increase tropospheric ozone and stratospheric water vapor content.[1] World wide-agriculture makes up one-fifth of the anthropogenic greenhouse effect and generates half of the total anthropogenic methane emissions.[10]
Reduction of Methane Emissions
One method of methane emission mitigation is the use of vaccines against rumen methanogens. Vaccines either lessen the activity of rumen methanogens or decrease their numbers, and would be more cost effective and manageable in livestock that graze. The general idea of a vaccine would include a significant salivary antibody response to introduce antibodies to the rumen and stop some methanogen function.[11] In a study conducted on methane emissions from sheep with vaccinations specific to certain species and strains of rumen methanogens, vaccines caused a serum antibody response which altered methanogen diversity and population. The vaccine also completely or partially eliminated some strains of Methanobrevibacter (a methanogen genus).[12]
Other methods of reducing methane emissions include the use of bacteriophages, homoacetogens, protozoa, and dry matter intake and nutrition. Bacteriophages’ ability to lyse host cells has proven an advantage helpful in curbing methanogenesis from specific methanogen species and strains. Bacteriophages, as is necessary for methanogen-reducing vaccines, specify particular host species or strains and when used to decrease methanogen diversity or populations, work against certain methanogens while leaving others undisturbed in the rumen. However, in order for an efficient use of phages against methanogens, many more phages must be sequenced in order to keep up with hosts, who evolve quickly in order to reduce their risk of infection. In addition, extreme phage specificity reduces the possibility of an effective methane decrease as many different methanogen species and strains exist in ruminants.[11]
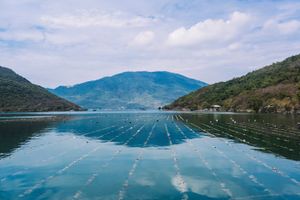
Homoacetogens are bacteria that grow using H2 as energy by reducing it to CO2 and then acetate. In order to reduce methane loss from ruminant feed energy and increase animal efficiency, H2 energy forms acetate and methane production would decrease. Normally, low amounts of H2 in rumen results in fewer homoacetogens than methanogens. By increasing the concentration of homoacetogens in the rumen, more H2 will be used by homoacetogens resulting in an overall methane reduction. Also related to H2 use is protozoa, which transfers H2 to methanogens. By removing or controlling rumen protozoa, less H2 would be present for use in methanogenesis. Possible implementations of minimizing protozoa numbers include breeding entire herds free of protozoa (often done in isolation), the use of detergents to reduce protozoa (chemical defaunation), the use of oils, saponins, and tannins, and focus on diet. Diet changes could potentially cause changes that create an inhospitable environment for protozoa, such as a fluctuation in pH. Another possible diet change is an increased consumption of soluble sugars, which generally produce less methane than plant fibers.[11]
One challenge with changing ruminant diets is the massive production rates necessary for changes to influence overall methane emissions. A recent study examined the effect of seaweed in cattle feed on methane emissions from cows. Research dealt mostly with the Asparagopsis taxiformis species from Southern California and the possibility of growing the plant on a scale large enough to be incorporated into cattle feed. The challenge, however, is growing seaweed in aquaculture farms, as most seaweed farming occurs in only a few Asian nations (Figure 5).[13]
Conclusion
Methanosarcina barkeri CM1 is one of many species of rumen methanogens. As the raising and consumption of ruminants increases, so too does the amount of food and water necessary to sustain this industry. Additionally, because the CM1 strain, similar to other methanogens, can produce methane from multiple molecules, decreasing methane production from animals used for food throughout history and the world proves extremely challenging. However, as global warming becomes an increasing threat, efforts are ongoing to find new ways to keep this food source available while reducing greenhouse gas emissions.
References
- ↑ 1.0 1.1 1.2 1.3 1.4 Hook, Sarah E et al. “Methanogens: methane producers of the rumen and mitigation strategies.” Archaea (Vancouver, B.C.) vol. 2010 945785. 30 Dec. 2010, doi:10.1155/2010/945785. https://www.ncbi.nlm.nih.gov/pmc/articles/PMC3021854/
- ↑ Jarvis, G., Strömpl, C., Burgess, D. et al. Isolation and Identification of Ruminal Methanogens from Grazing Cattle. Curr Microbiol 40, 327–332 (2000).[1]
- ↑ 3.00 3.01 3.02 3.03 3.04 3.05 3.06 3.07 3.08 3.09 3.10 Lambie, S.C., Kelly, W.J., Leahy, S.C. et al. The complete genome sequence of the rumen methanogen Methanosarcina barkeri CM1. Stand in Genomic Sci 10, 57 (2015). [2]
- ↑ 4.0 4.1 Owens F.N., Basalan M. (2016) Ruminal Fermentation. In: Millen D., De Beni Arrigoni M., Lauritano Pacheco R. (eds) Rumenology. Springer, Cham. https://doi.org/10.1007/978-3-319-30533-2_3 [3]
- ↑ Libretexts. “1.3: Bacterial Restriction/Modification System.” Biology LibreTexts, 14 July 2020.
- ↑ Maeder, Dennis L., et al. “The Methanosarcina Barkeri Genome: Comparative Analysis with Methanosarcina Acetivorans and Methanosarcina Mazei Reveals Extensive Rearrangement within Methanosarcinal Genomes.” Journal of Bacteriology, American Society for Microbiology Journals, 15 Nov. 2006, jb.asm.org/content/188/22/7922.
- ↑ 7.0 7.1 Rohlin, Lars, et al. “Identification of the Major Expressed S-Layer and Cell Surface-Layer-Related Proteins in the Model Methanogenic Archaea: Methanosarcina Barkeri Fusaro and Methanosarcina Acetivorans C2A.” Archaea, Hindawi, 15 May 2012, www.hindawi.com/journals/archaea/2012/873589/.
- ↑ 8.0 8.1 Feist, Adam M, Scholten, Johannes C M, Palsson, Bernhard Ø, Brockman, Fred J, Ideker, Trey, (2006) Modeling methanogenesis with a genome‐scale metabolic reconstruction of Methanosarcina barkeri. Molecular Systems Biology, 2. 2006.0004. doi: accession:10.1038/msb4100046[4]
- ↑ Gonnerman, M.C., Benedict, M.N., Feist, A.M., Metcalf, W.W. and Price, N.D. (2013), Genomically and biochemically accurate metabolic reconstruction of Methanosarcina barkeri Fusaro, iMG746. Biotechnology Journal, 8: 1070-1079.[5]
- ↑ Ellis, J. L., et al. “Prediction of Methane Production from Dairy and Beef Cattle.” Journal of Dairy Science, vol. 90, no. 7, 2007, pp. 3456–66. Crossref, doi:10.3168/jds.2006-675.[6]
- ↑ 11.0 11.1 11.2 Buddle, Bryce M., et al. “Strategies to Reduce Methane Emissions from Farmed Ruminants Grazing on Pasture.” The Veterinary Journal, vol. 188, no. 1, 2011, pp. 11–17. Crossref, doi:10.1016/j.tvjl.2010.02.019.[7]
- ↑ Williams, Yvette J., et al. “A Vaccine against Rumen Methanogens Can Alter the Composition of Archaeal Populations.” Applied and Environmental Microbiology, vol. 75, no. 7, 2009, pp. 1860–66. Crossref, doi:10.1128/aem.02453-08.[8]
- ↑ Wozniacka, Gosia. “Can We Grow Enough Seaweed to Help Cows Fight Climate Change?” Civil Eats, 19 Sept. 2019, civileats.com/2019/06/03/can-we-grow-enough-seaweed-to-help-cows-fight-climate-change.[9]
Edited by Emily Banthin, student of Joan Slonczewski for BIOL 116 Information in Living Systems, 2020, Kenyon College.