Comamonas badia: Difference between revisions
No edit summary |
|||
(49 intermediate revisions by the same user not shown) | |||
Line 1: | Line 1: | ||
{{Uncurated}} | {{Uncurated}} | ||
[[Image:Electron_micrograph_of_Comamonas_badia.jpeg|thumbnail|Figure 1. Electron micrograph of ''Comamonas badia'' with the visible single flagellum. Source: [http://bioenergyrus.blogspot.ca/2008/05/what-floc.html Bioenergyrus blog]]] | |||
=Classification= | =Classification= | ||
==Higher Order Taxa== | ==Higher Order Taxa== | ||
Bacteria (Kingdom), Proteobacteria (Phylum), Betaproteobacteria (Class), Burkholderiales (Order), Comamonadaceae (Family), Comamonas (Genus) | Bacteria (Kingdom), Proteobacteria (Phylum), Betaproteobacteria (Class), Burkholderiales (Order), Comamonadaceae (Family), [http://en.wikipedia.org/wiki/Comamonas ''Comamonas''](Genus) | ||
==Species== | ==Species== | ||
Line 12: | Line 15: | ||
=Description= | =Description= | ||
[[Image: | [[Image:"Flocculent_culture_of_Comamonas_and_Nitrosomonas.jpeg|thumb|250px|right|Figure 2. Flocculent culture of ''Comamonas'' and ''Nitrosomonas''. Interaction between the two species is still under investigation. Source: [http://bioenergyrus.blogspot.ca/2008/05/what-floc.html Bioenergyrus blog]]] | ||
''Comamonas badia'' is a gram negative, rod-shaped, strictly aerobic, and highly motile bacteria with a polar flagellum. It was isolated from phenol-adapted [http://en.wikipedia.org/wiki/Activated_sludge activated sludge] from a factory sludge from Japan [[#References|[1]]]. It was originally classified under the genus ''Pseudomonas'' [[#References|[3]]][[#References|[4]]]. Based on 16S rRNA gene sequence and G+C content, ''C. badia'' was determined to represent its own genus, ''Comamonas''. This novel one was first represented by the species ''C. badia'', type-strain IAM 14839<sup>T</sup> [[#References|[1]]][[#References|[9]]]. | |||
''Comamonas badi'' forms pink colonies when cultured in Luria slant media and forms visible [http://en.wikipedia.org/wiki/Flocculation flocs] in Luria broth media, unlike other Comamonas species which do not form flocs [[#References|[2]]]. ''Comamonas badia'' grows at a very slow rate in a flocculated state with a generation time of about 12 hours at optimal temperatures (27-30°C) in LB media and produces few free-floating cells. When cultured in broths, the supernatant is usually clear [[#References|[4]]]. This bacterium grows the slowest at 20°C (19 hours for generation time) and does not grow at 37°C (average human body temperature), unlike other ''Comamonas'' species which do undergo growth at 37°C [[#References|[1]]]. For floc formation, ''C. badia'' grows embedded in a film mesh structure made out of [http://en.wikipedia.org/wiki/Glycosaminoglycan mucopolysaccharides] which consists of glucosamine, glucose, mannose, galactose and rhamnose. Only about 10% of the total polysaccharide content found with ''C. badia'' is involved with floc formation [[#References|[3]]]. The ability to form flocs gives this species a protection advantage from predators compared to free roaming cells, allows other bacteria to form on the flocs, and prevents the organism from being washed out of the system, i.e. activated sludge [[#References|[10]]]. | |||
=Significance= | |||
It has been found that ''C. badia'' has moderate [http://en.wikipedia.org/wiki/Phenol phenol] tolerance and moderate phenol degradation capabilities. ''C. badia'' existing in a floc-formation is also the most predominating bacteria species in activated sludge and is involved in degrading highly toxic and carcinogenic phenol compounds in [http://en.wikipedia.org/wiki/Coke_(fuel) coke] wastewater [[#References|[2]]]. Being able to tolerate and degrade such toxic compounds, the ability to form flocs is an integral part this microbe in activated sludge to treat wastewaters [[#References|[10]]]. ''C. badia'' has a unique ability in which it is responsible for its own deflocculation by producing an [http://academic.pgcc.edu/~kroberts/web/exoenzymes/exoenzymes.htm exoenzyme]. Various commercial enzymes are unable to induce deflocculation in ''C. badia'' and therefore the bacteria’s life activities are determined by their own accord via polysaccharide-synthesizing enzymes and deflocculating enzymes [[#References|[5]]]. | |||
= | =Phylogenetics= | ||
[[ | [[Image:Phylogenic_Tree_of_Comamonas_badia.jpeg|thumb|300px|left|Figure 3. Simplified phylogenetic tree made comparing 16S rDNA sequence of ''Comamonas badia'' to some members of the β-proteobacteria. ''Alcaligenes faecalis'' is used as an outgroup. Source: Image is derived from ''Fig. 2'' from Tago Y. and Yokota A. (2004) [[#References|[1]]]] | ||
It was only recently in 2004 that ''C. badia'' was introduced and recognized as a novel species, using comparative analysis of almost complete [http://en.wikipedia.org/wiki/16S_rDNA 16S rDNA] gene sequence and [http://en.wikipedia.org/wiki/GC-content G+C content] of DNA on the strain IAM 14839<sup>T</sup>. The complete genome of ''C. badia'' has yet to be sequenced [[#References|[1]]][[#References|[9]]]. | |||
= | =Cell Structure= | ||
[[ | ''C. badia'' are gram negative, highly motile, 0.8-0.9 × 1.0-2.5 μm, and rod-shaped bacterium that appears in singles or pairs in young cultures. They grow very slowly in a flocculated state (12+ hours for doubling time) and have a single polar flagellum for motility. ''C. badia'' are catalase and oxidase-positive, heterotrophic organisms that do not use glucose, mannose, arabinose, or sucrose for growth, but do use glutamic acid or aspartic acid and is able to reduce nitrate [[#References|[1]]][[#References|[6]]]. They are capable of growing in cultures without growth factors and do not hydrolyze gelatin. ''C. badia'' contains the [http://books.google.ca/books?id=9cwgo-9IyTUC&pg=PA609&lpg=PA609&dq=ubiquinone+q-8&source=bl&ots=lRwaaltf0C&sig=O4GJr1aWLkt01wxTIF6xULgKvuE&hl=en&sa=X&ei=xEfJUO7GJK_uigK-qIDwCw&sqi=2&ved=0CHEQ6AEwCA#v=onepage&q=ubiquinone%20q-8&f=false ubiquinone Q-8] as the predominant component of the [http://en.wikipedia.org/wiki/Quinone quinone] system for electron transport. ''Comamonas badia'' is largely composed of [http://en.wikipedia.org/wiki/Exopolysaccharide exopolysaccharides] (EPS), containing large amounts of straight-chain unsaturated fatty acids, cis-7-hexadecenoic acid (16:1ω7c) and saturated fatty acid, hexadecanoic acid (16:0) [[#References|[1]]]. Large amounts of these EPS contribute to the high EPS to protein ratio, which are commonly found in bacteria within activated sludge [[#References|[7]]]. This ratio also gives the flocs formed by this microbe a high hydrophobic and negative charge characteristic. A high hydrophobic property helps microbes form flocs easier and is normally affected by growth rate as well [[#References|[7]]][[#References|[8]]]. | ||
=Ecology= | |||
''C. badia'' was isolated and found in phenol-adapted activated sludge in a factory plant in Japan used for decomposing industrial wastes. This species is able to thrive in such environments not only due to its ability to degrade phenols but also its ability to grow and tolerate an environment with up to 500 mg/L of phenols. It may have a prominent role in structure formation of the activated sludge ecology as ''C. badia'' is the most predominate species in activated sludge, roughly 32-66% of total peak area of the community [[#References|[2]]]. ''C. badia'' can also form a productive interaction with phenol-decomposing bacteria Strain No. 3 from the activated sludge to greatly enhance phenol degradation and floc formation abilities. High phenol-decomposing bacteria such as Strain No. 3 requires floc formation or floc incorporation in order to degrade phenols efficiently. Unable to form flocs on its own, Strain No. 3 is usually incorporated with ''C. badia'' and is the only strain that is able to from a floc formation with ''C. badia''. This combination of Strain No. 3 and ''C. badia'' in a ratio of 1:6, respectively, in a floc is able to decompose phenol concentrations as high as 700 ppm rapidly without deflocculating for over 50 hours. However, Strain No. 3 bacteria must be incorporated into the ''C. badia'' floc rather than interacting with the floc as free roaming cells. The latter method will result with displays of slow and non-effective phenol decomposition and deflocculation after the 48 hour incubation point. Strain No. 3 and ''C. badia'' may have a nutritional interaction and ''C. badia'' may provide a structural advantage for Strain No.3 as a floc allowing such combination to decompose high levels of phenol with the presence of peptone [[#References|[4]]]. Such positive interaction between the bacteria may provide useful environmental interaction in decomposing harmful toxic substances such as phenols in industrial wastewater such as coke wastewater [[#References|[2]]]. | |||
= | =Unique Deflocculation of ''Comamonas badia''= | ||
[[ | [[Image:Original_pic1_of_pH_on_Enzyme.jpeg|thumb|400px|right|Figure 4. Graph displaying the effect that pH has on the enzymatic activity of the polysaccharide hydrolase exoenzyme that ''Comamonas badia'' produces. Deflocculation occurs around pH 7 while deflocculation occurs around pH 3.8-5.0. Source: Image derived from ''Fig. 5'' from Tago Y. and Aida K. (1975) [[#References|[5]]]] | ||
Not only is ''C. badia'' capable of producing its own exoenzyme to induce deflocculation, it also has the ability to resist deflocculation by various commercial cellulases, proteases, lysozymes and EDTA. However, the species lose this resisting ability if the cell cultures were dialyzed before treatment with commercial enzymes. The self produced exoenzyme, which is an exopolymer-degrading enzyme or other known as a [http://www.researchgate.net/publication/5280441_Polysaccharide_hydrolase_folds_diversity_of_structure_and_convergence_of_function polysaccharide hydrolase], has been isolated and purified to test its functions and characteristics. When the activity of hydrolysis of polymers is higher than the synthesis of polymers, deflocculation occurs. The optimal pH for deflocculation is around the range of 3.8 – 5.0, which is far from the normal pH of flocculation (7.0). At normal flocculation pH, only less than 10% of enzymatic activity was observed from the hydrolase. The exoenzyme maintains stable conformation in normal temperatures until 35°C and becomes completely inactive if left at a temperature of 45°C for 5 minutes. The exoenzyme’s activity is also inhibited at buffer ionic strengths higher than 0.01, and in mixtures of basic or amino acids at concentrations higher than 10 mM. Amino sugars and uronic acids also inhibits the enzyme at 10 mM concentrations. Glucosamine however, effectively inhibits enzymatic activity at 1 mM concentrations. Although this powerful enzyme was able to induce ''C. badia'' deflocculation by degrading and releasing sugars, it cannot hydrolyze cellulose, pectin, or ethylene glycol chitin [[#References|[5]]]. | |||
=References= | =References= | ||
(1) Tago Y., Yokota A. | (1) [http://www.ncbi.nlm.nih.gov/pubmed/15747228 Tago Y., Yokota A. “''Comamonas badia'' sp nov., a Floc-forming Bacterium Isolated from Activated Sludge.” Journal of General and Applied Microbiology, 2004, DOI: 10.2323/jgam.50.243] | ||
(2) Felföldi T., Székely A., Gorál R., Barkács K., Scheirich G., András J, Rácz A., Márialigeti K. “Polyphasic Bacterial Community Analysis of an Aerobic Activated Sludge Removing Phenols and Thiocyanate from Coke Plant Effluent.” Bioresource Technology, 2010, DOI: 10.1016/j.biortech.2009.12.053 | (2) [http://www.ncbi.nlm.nih.gov/pubmed/20093025 Felföldi T., Székely A., Gorál R., Barkács K., Scheirich G., András J, Rácz A., Márialigeti K. “Polyphasic Bacterial Community Analysis of an Aerobic Activated Sludge Removing Phenols and Thiocyanate from Coke Plant Effluent.” Bioresource Technology, 2010, DOI: 10.1016/j.biortech.2009.12.053] | ||
(3) Tago Y., Aida K. “Exocellular Mucopolysaccharide Closely Related to Bacterial Floc Formation” Applied and Environmental Microbiology, 1977, ISSN: 0099-2240 | (3) [http://www.ncbi.nlm.nih.gov/pmc/articles/PMC242648/ Tago Y., Aida K. “Exocellular Mucopolysaccharide Closely Related to Bacterial Floc Formation” Applied and Environmental Microbiology, 1977, ISSN: 0099-2240] | ||
(4) Tago Y., Kuraishi H., Aida K. “The Formation of a Model Floc able to Decompose Phenol by the Mixed Culture of Bacteria Isolated from Activated Sludge.” Journal of General and Applied Microbiology, 1975, DOI: 10.2323/jgam.21.41 | (4) [https://www.jstage.jst.go.jp/article/jgam1955/21/1/21_1_41/_pdf Tago Y., Kuraishi H., Aida K. “The Formation of a Model Floc able to Decompose Phenol by the Mixed Culture of Bacteria Isolated from Activated Sludge.” Journal of General and Applied Microbiology, 1975, DOI: 10.2323/jgam.21.41] | ||
(5) Tago Y., Aida K. “The Deflocculating Enzyme Produced by a Floc-Forming Bacterium.” Journal of General and Applied Microbiology, 1975, DOI: 10.2323/jgam.21.365 | (5) [https://www.jstage.jst.go.jp/article/jgam1955/21/6/21_6_365/_pdf Tago Y., Aida K. “The Deflocculating Enzyme Produced by a Floc-Forming Bacterium.” Journal of General and Applied Microbiology, 1975, DOI: 10.2323/jgam.21.365] | ||
(6) Blöthe M., Roden E. “Composition and Activity of an Autotrophic Fe(II)-Oxidizing, Nitrate-Reducing Enrichment Culture” Applied and Environmental Microbiology, 2009, DOI: 10.1128/AEM.01742-09 | (6) [http://aem.asm.org/content/75/21/6937.full Blöthe M., Roden E. “Composition and Activity of an Autotrophic Fe(II)-Oxidizing, Nitrate-Reducing Enrichment Culture” Applied and Environmental Microbiology, 2009, DOI: 10.1128/AEM.01742-09] | ||
(7) Mahendran B., Lishman L., Liss S. “Structural, Physicochemical and Microbial Properties of Flocs and Biofilms in Integrated Fixed-Film Activated Sludge (IFFAS) Systems” Water Research (Oxford), 2012, DOI: 10.1016/j.watres.2012.05.058 | (7) [http://www.ncbi.nlm.nih.gov/pubmed/22832219 Mahendran B., Lishman L., Liss S. “Structural, Physicochemical and Microbial Properties of Flocs and Biofilms in Integrated Fixed-Film Activated Sludge (IFFAS) Systems” Water Research (Oxford), 2012, DOI: 10.1016/j.watres.2012.05.058] | ||
(8) Zita A., Hermansson M. “Effects of Bacterial Cell Surface Structures and Hydrophobicity on Attachment of Activated Sludge Flocs” Applied and Environmental Microbiology, 1997, ISSN: 0099-2240 | (8) [http://www.ncbi.nlm.nih.gov/pmc/articles/PMC168408/ Zita A., Hermansson M. “Effects of Bacterial Cell Surface Structures and Hydrophobicity on Attachment of Activated Sludge Flocs” Applied and Environmental Microbiology, 1997, ISSN: 0099-2240] | ||
(9) Stackebrandt E., Goebel B. “Taxonomic Note: A place for DNA-DNA Reassociation and 16S rRNA Sequence Analysis in the Present Species Definition in Bacteriology” International Journal of Systematic Bacteriology, 1994, ISSN: 0020-7713 | (9) [http://ijs.sgmjournals.org/content/44/4/846.abstract Stackebrandt E., Goebel B. “Taxonomic Note: A place for DNA-DNA Reassociation and 16S rRNA Sequence Analysis in the Present Species Definition in Bacteriology” International Journal of Systematic Bacteriology, 1994, ISSN: 0020-7713] | ||
(10) Raszka A., Chorvatova M., Wanner J. “The Role and Significance of Extracellular Polymers in Activated Sludge. Part I: Literature Review” Acta Hydrochimica et Hydrobiologica (Weinheim an der Bergstrasse, Germany), 2006, DOI: 10.1002/aheh.200500640 | (10) [http://onlinelibrary.wiley.com/doi/10.1002/aheh.200500640/abstract Raszka A., Chorvatova M., Wanner J. “The Role and Significance of Extracellular Polymers in Activated Sludge. Part I: Literature Review” Acta Hydrochimica et Hydrobiologica (Weinheim an der Bergstrasse, Germany), 2006, DOI: 10.1002/aheh.200500640] |
Latest revision as of 21:30, 15 January 2013
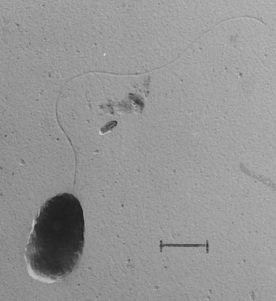
Classification
Higher Order Taxa
Bacteria (Kingdom), Proteobacteria (Phylum), Betaproteobacteria (Class), Burkholderiales (Order), Comamonadaceae (Family), Comamonas(Genus)
Species
Comamonas badia
Description
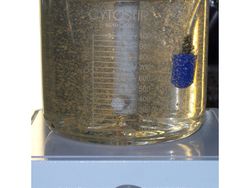
Comamonas badia is a gram negative, rod-shaped, strictly aerobic, and highly motile bacteria with a polar flagellum. It was isolated from phenol-adapted activated sludge from a factory sludge from Japan [1]. It was originally classified under the genus Pseudomonas [3][4]. Based on 16S rRNA gene sequence and G+C content, C. badia was determined to represent its own genus, Comamonas. This novel one was first represented by the species C. badia, type-strain IAM 14839T [1][9].
Comamonas badi forms pink colonies when cultured in Luria slant media and forms visible flocs in Luria broth media, unlike other Comamonas species which do not form flocs [2]. Comamonas badia grows at a very slow rate in a flocculated state with a generation time of about 12 hours at optimal temperatures (27-30°C) in LB media and produces few free-floating cells. When cultured in broths, the supernatant is usually clear [4]. This bacterium grows the slowest at 20°C (19 hours for generation time) and does not grow at 37°C (average human body temperature), unlike other Comamonas species which do undergo growth at 37°C [1]. For floc formation, C. badia grows embedded in a film mesh structure made out of mucopolysaccharides which consists of glucosamine, glucose, mannose, galactose and rhamnose. Only about 10% of the total polysaccharide content found with C. badia is involved with floc formation [3]. The ability to form flocs gives this species a protection advantage from predators compared to free roaming cells, allows other bacteria to form on the flocs, and prevents the organism from being washed out of the system, i.e. activated sludge [10].
Significance
It has been found that C. badia has moderate phenol tolerance and moderate phenol degradation capabilities. C. badia existing in a floc-formation is also the most predominating bacteria species in activated sludge and is involved in degrading highly toxic and carcinogenic phenol compounds in coke wastewater [2]. Being able to tolerate and degrade such toxic compounds, the ability to form flocs is an integral part this microbe in activated sludge to treat wastewaters [10]. C. badia has a unique ability in which it is responsible for its own deflocculation by producing an exoenzyme. Various commercial enzymes are unable to induce deflocculation in C. badia and therefore the bacteria’s life activities are determined by their own accord via polysaccharide-synthesizing enzymes and deflocculating enzymes [5].
Phylogenetics
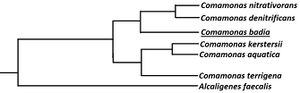
It was only recently in 2004 that C. badia was introduced and recognized as a novel species, using comparative analysis of almost complete 16S rDNA gene sequence and G+C content of DNA on the strain IAM 14839T. The complete genome of C. badia has yet to be sequenced [1][9].
Cell Structure
C. badia are gram negative, highly motile, 0.8-0.9 × 1.0-2.5 μm, and rod-shaped bacterium that appears in singles or pairs in young cultures. They grow very slowly in a flocculated state (12+ hours for doubling time) and have a single polar flagellum for motility. C. badia are catalase and oxidase-positive, heterotrophic organisms that do not use glucose, mannose, arabinose, or sucrose for growth, but do use glutamic acid or aspartic acid and is able to reduce nitrate [1][6]. They are capable of growing in cultures without growth factors and do not hydrolyze gelatin. C. badia contains the ubiquinone Q-8 as the predominant component of the quinone system for electron transport. Comamonas badia is largely composed of exopolysaccharides (EPS), containing large amounts of straight-chain unsaturated fatty acids, cis-7-hexadecenoic acid (16:1ω7c) and saturated fatty acid, hexadecanoic acid (16:0) [1]. Large amounts of these EPS contribute to the high EPS to protein ratio, which are commonly found in bacteria within activated sludge [7]. This ratio also gives the flocs formed by this microbe a high hydrophobic and negative charge characteristic. A high hydrophobic property helps microbes form flocs easier and is normally affected by growth rate as well [7][8].
Ecology
C. badia was isolated and found in phenol-adapted activated sludge in a factory plant in Japan used for decomposing industrial wastes. This species is able to thrive in such environments not only due to its ability to degrade phenols but also its ability to grow and tolerate an environment with up to 500 mg/L of phenols. It may have a prominent role in structure formation of the activated sludge ecology as C. badia is the most predominate species in activated sludge, roughly 32-66% of total peak area of the community [2]. C. badia can also form a productive interaction with phenol-decomposing bacteria Strain No. 3 from the activated sludge to greatly enhance phenol degradation and floc formation abilities. High phenol-decomposing bacteria such as Strain No. 3 requires floc formation or floc incorporation in order to degrade phenols efficiently. Unable to form flocs on its own, Strain No. 3 is usually incorporated with C. badia and is the only strain that is able to from a floc formation with C. badia. This combination of Strain No. 3 and C. badia in a ratio of 1:6, respectively, in a floc is able to decompose phenol concentrations as high as 700 ppm rapidly without deflocculating for over 50 hours. However, Strain No. 3 bacteria must be incorporated into the C. badia floc rather than interacting with the floc as free roaming cells. The latter method will result with displays of slow and non-effective phenol decomposition and deflocculation after the 48 hour incubation point. Strain No. 3 and C. badia may have a nutritional interaction and C. badia may provide a structural advantage for Strain No.3 as a floc allowing such combination to decompose high levels of phenol with the presence of peptone [4]. Such positive interaction between the bacteria may provide useful environmental interaction in decomposing harmful toxic substances such as phenols in industrial wastewater such as coke wastewater [2].
Unique Deflocculation of Comamonas badia

Not only is C. badia capable of producing its own exoenzyme to induce deflocculation, it also has the ability to resist deflocculation by various commercial cellulases, proteases, lysozymes and EDTA. However, the species lose this resisting ability if the cell cultures were dialyzed before treatment with commercial enzymes. The self produced exoenzyme, which is an exopolymer-degrading enzyme or other known as a polysaccharide hydrolase, has been isolated and purified to test its functions and characteristics. When the activity of hydrolysis of polymers is higher than the synthesis of polymers, deflocculation occurs. The optimal pH for deflocculation is around the range of 3.8 – 5.0, which is far from the normal pH of flocculation (7.0). At normal flocculation pH, only less than 10% of enzymatic activity was observed from the hydrolase. The exoenzyme maintains stable conformation in normal temperatures until 35°C and becomes completely inactive if left at a temperature of 45°C for 5 minutes. The exoenzyme’s activity is also inhibited at buffer ionic strengths higher than 0.01, and in mixtures of basic or amino acids at concentrations higher than 10 mM. Amino sugars and uronic acids also inhibits the enzyme at 10 mM concentrations. Glucosamine however, effectively inhibits enzymatic activity at 1 mM concentrations. Although this powerful enzyme was able to induce C. badia deflocculation by degrading and releasing sugars, it cannot hydrolyze cellulose, pectin, or ethylene glycol chitin [5].