Megavirales Genetics from Mimivirus to Chilensis: Difference between revisions
No edit summary |
No edit summary |
||
Line 25: | Line 25: | ||
<br> | <br> | ||
Every point of information REQUIRES CITATION using the citation tool shown above. | Every point of information REQUIRES CITATION using the citation tool shown above. | ||
==Support For Megavirales as a Fourth Domain== | ==Support For Megavirales as a Fourth Domain== | ||
<br>The classification of NCLDVs as a defining phylogenetic group, or the establishment of Megavirales as a proposed new order is one that does not go unchallenged in the face of certain objections to phylogenies created from viral DNA. This is for two main reasons: Viruses evolve at an extremely rapid rate meaning that consistency will be difficult to maintain from one genetic analysis to the next, within a small set of constantly changing genes. The second reason is that viruses are liable to engage regularly in horizontal gene transfer with the host muddying up clear relationships between species or patterns of descent. However, in the case of NCLDVs these objections are less applicable. These double-stranded DNA viruses do not evolve at a rate comparable to smaller viruses with homologs that are significantly conserved, therefore we might expect the significant difference between NCLDVs to be a result of divergence that has occurred over a significant amount of time, suggesting a common ancestor for NCLDVs. The idea that horizontal gene transfer occurs regularly is also challenged in Megaviruses, due to their ability to rely completely on the cellular processes of the host. Therefore, there is not much evolutionary incentive for them to retain host genomes in NCLDV populations, in fact the pressure would suggest more static gene populations, or further reductive evolution, barring some unforeseen environmental shift. There is also not genetic evidence for clear-cut examples of gene transfer that has occurred between Eukaryotic hosts and their associated NCLDVs. For example, the open reading frames for non-homologous genes between Megavirus and MImivirus are 80% different, much higher than would be expected in the case of horizontal gene transfer. However, certain sets of genes (described further in gene product section) are sufficiently conserved in order to facilitate and form an adequate phylogeny (Legendre, 2012). To build a phylogeny, the Legendre paper used two specific genes that had excellent conservation in NCLDV’s and that were necessary for DNA replication. The first being a ribonucleoside diphosphate reductase sub-unit and a DNA replication clamp loader called factor C. This analysis illustrates that these genes show clear distinction from Archaea and Eukaryotes, showing little, or no overlapping affinities between these orders. Said another way, Megavirales as a proposed order, appears just as separate from Eukaryotes as Archaea are. Also up for speculation is whether or not Megavirales are at the root of the Eukaryotic domain as in Figure 2A, or are not evolutionary pre-cursors to Eukaryotes as in Fig. 2B. | <br>The classification of NCLDVs as a defining phylogenetic group, or the establishment of Megavirales as a proposed new order is one that does not go unchallenged in the face of certain objections to phylogenies created from viral DNA. This is for two main reasons: Viruses evolve at an extremely rapid rate meaning that consistency will be difficult to maintain from one genetic analysis to the next, within a small set of constantly changing genes. The second reason is that viruses are liable to engage regularly in horizontal gene transfer with the host muddying up clear relationships between species or patterns of descent. However, in the case of NCLDVs these objections are less applicable. These double-stranded DNA viruses do not evolve at a rate comparable to smaller viruses with homologs that are significantly conserved, therefore we might expect the significant difference between NCLDVs to be a result of divergence that has occurred over a significant amount of time, suggesting a common ancestor for NCLDVs. The idea that horizontal gene transfer occurs regularly is also challenged in Megaviruses, due to their ability to rely completely on the cellular processes of the host. Therefore, there is not much evolutionary incentive for them to retain host genomes in NCLDV populations, in fact the pressure would suggest more static gene populations, or further reductive evolution, barring some unforeseen environmental shift. There is also not genetic evidence for clear-cut examples of gene transfer that has occurred between Eukaryotic hosts and their associated NCLDVs. For example, the open reading frames for non-homologous genes between Megavirus and MImivirus are 80% different, much higher than would be expected in the case of horizontal gene transfer. However, certain sets of genes (described further in gene product section) are sufficiently conserved in order to facilitate and form an adequate phylogeny (Legendre, 2012). To build a phylogeny, the Legendre paper used two specific genes that had excellent conservation in NCLDV’s and that were necessary for DNA replication. The first being a ribonucleoside diphosphate reductase sub-unit and a DNA replication clamp loader called factor C. This analysis illustrates that these genes show clear distinction from Archaea and Eukaryotes, showing little, or no overlapping affinities between these orders. Said another way, Megavirales as a proposed order, appears just as separate from Eukaryotes as Archaea are. Also up for speculation is whether or not Megavirales are at the root of the Eukaryotic domain as in Figure 2A, or are not evolutionary pre-cursors to Eukaryotes as in Fig. 2B. | ||
Line 30: | Line 31: | ||
==Sequencing Methods== | ==Sequencing Methods== | ||
[[Image:Roche sequencing.gif|thumb| | [[Image:Roche sequencing.gif|thumb|150px|right|Figure 4: A summary and visualization of Roche-454 Pyrosequencing methods(http://www.annualreviews.org/na101/home/literatum/publisher/ar/journals/content/genom/2008/genom.2008.9.issue-1/annurev.genom.9.081307.164359/production/images/medium/gg090387.f1.gif]] | ||
<br>Roche-454 Pyrosequencing was one of the first methods to gain commercial use for rapid genetic sequencing and is in common use for obtaining the phylogeny analyses discussed earlier. Pyrosequencing is powered by the release of pyrophosphates at every nucleotide addition. This pyrophosphate release stimulates a cascade that results in the eventual light production by firefly enzyme luciferase. The measured light correlates to number of base pairs that have been constructed. (Mardis, 2008). First DNA is separated to form an ssDNA library by way of bonding to a multitude of agarose beads containing complementary oligonucleotide sequences. Then these beads are separated in mixtures of oil and water that isolate each bead in micelles with PCR reactants. This means that each bead ends up with millions of copies of a single strand of DNA. Each bead is then arranged and fixed in place by the wells of a picotiter plate. Agarose beads are then surrounded by enzyme-containing beads that are responsible for catalyzing associated reactions that facilitate nucleotide addition through the creation of downstream pathways. The isolated addition in all wells is read simultaneously by CCD cameras, as pure nucleotide solution is added. The emitted light allows the camera to tell which nucleotides are added and computer analysis may then align complementary strands to generate a whole sequence. This process affords 250 nucleotides per read, and the computer eliminates the presence of mixed beads with mixed initial ssDNA’s, or beads with DNA that is not affixed and is lacking the calibratory TCGA sequence. This system is not perfect however, due to the manner in which pyrosequencing is calibrated, reads of the same base over 6 times lead to a particular kind of error, although other errors are certainly possible, this is where other methods of sequencing can fill in the gaps.<br> | <br>Roche-454 Pyrosequencing was one of the first methods to gain commercial use for rapid genetic sequencing and is in common use for obtaining the phylogeny analyses discussed earlier. Pyrosequencing is powered by the release of pyrophosphates at every nucleotide addition. This pyrophosphate release stimulates a cascade that results in the eventual light production by firefly enzyme luciferase. The measured light correlates to number of base pairs that have been constructed. (Mardis, 2008). First DNA is separated to form an ssDNA library by way of bonding to a multitude of agarose beads containing complementary oligonucleotide sequences. Then these beads are separated in mixtures of oil and water that isolate each bead in micelles with PCR reactants. This means that each bead ends up with millions of copies of a single strand of DNA. Each bead is then arranged and fixed in place by the wells of a picotiter plate. Agarose beads are then surrounded by enzyme-containing beads that are responsible for catalyzing associated reactions that facilitate nucleotide addition through the creation of downstream pathways. The isolated addition in all wells is read simultaneously by CCD cameras, as pure nucleotide solution is added. The emitted light allows the camera to tell which nucleotides are added and computer analysis may then align complementary strands to generate a whole sequence. This process affords 250 nucleotides per read, and the computer eliminates the presence of mixed beads with mixed initial ssDNA’s, or beads with DNA that is not affixed and is lacking the calibratory TCGA sequence. This system is not perfect however, due to the manner in which pyrosequencing is calibrated, reads of the same base over 6 times lead to a particular kind of error, although other errors are certainly possible, this is where other methods of sequencing can fill in the gaps.<br> | ||
[[Image:Illumina sequencing.gif|thumb| | [[Image:Illumina sequencing.gif|thumb|150px|right|Figure 5: Illumina visual summary(http://www.annualreviews.org/na101/home/literatum/publisher/ar/journals/content/genom/2008/genom.2008.9.issue-1/annurev.genom.9.081307.164359/production/images/medium/gg090387.f2.gif]] | ||
<br>The other method occasionally used in conjunction with Roche 454 pyrosequencing is a combination of Illumina and Sanger techniques(Arslan, 2011). For Illumina sequencing DNA is transected randomly and bound to Illumina adapter sequences at each end of DNA. These adapter sequences tether to oligonucleotide flow cells and complementary adapter strands bind together to form bridges that facilitate repeated amplification of DNA at flow cell clusters. Eventually strands are replicated in clusters of optimally a million identical DNA strands similar to the Roche agarose clusters and then they are separated. Then to finally sequence the data the addition of differential ACGT fluorescent nucleotides is imaged after each unique fluorescent base binds one at a time. Then the data is analyzed for read quality, and any bad reads are discarded. Random sequences of 25-35 base pairs are assessed for similarity and overlapped according to repeat sequences. This level of automation constructed in sequencing genetic information is takes up large amounts of data storage and requires a relatively powerful computer for the sequencing software algorithms, but the time and money saved as a result as revolutionized the research that can be done in genetic analysis. It is now possible to gain detailed and potentially fruitful information about the proteins coded for by the isolates of novel microbe genomes, such as those of NCLDVs.<br> | <br>The other method occasionally used in conjunction with Roche 454 pyrosequencing is a combination of Illumina and Sanger techniques(Arslan, 2011). For Illumina sequencing DNA is transected randomly and bound to Illumina adapter sequences at each end of DNA. These adapter sequences tether to oligonucleotide flow cells and complementary adapter strands bind together to form bridges that facilitate repeated amplification of DNA at flow cell clusters. Eventually strands are replicated in clusters of optimally a million identical DNA strands similar to the Roche agarose clusters and then they are separated. Then to finally sequence the data the addition of differential ACGT fluorescent nucleotides is imaged after each unique fluorescent base binds one at a time. Then the data is analyzed for read quality, and any bad reads are discarded. Random sequences of 25-35 base pairs are assessed for similarity and overlapped according to repeat sequences. This level of automation constructed in sequencing genetic information is takes up large amounts of data storage and requires a relatively powerful computer for the sequencing software algorithms, but the time and money saved as a result as revolutionized the research that can be done in genetic analysis. It is now possible to gain detailed and potentially fruitful information about the proteins coded for by the isolates of novel microbe genomes, such as those of NCLDVs.<br> | ||
Line 39: | Line 40: | ||
[[Image:Mimivirus replication.jpg|thumb|200px|right|Figure 6: The Mimivirus replication cycle visualized with Transmission Electron Microscopy(http://ars.els-cdn.com/content/image/1-s2.0-S0168952510001460-gr1.jpg]] | [[Image:Mimivirus replication.jpg|thumb|200px|right|Figure 6: The Mimivirus replication cycle visualized with Transmission Electron Microscopy(http://ars.els-cdn.com/content/image/1-s2.0-S0168952510001460-gr1.jpg]] | ||
[[Image:Viral Factory.jpg|thumb|200px|left|Figure 7: Visualization of Volcanic-Like Viral Factories with DIC and DAPI staining(http://www.ncbi.nlm.nih.gov/pmc/articles/PMC1828621/bin/pone.0000328.g005.jpg]] | [[Image:Viral Factory.jpg|thumb|200px|left|Figure 7: Visualization of Volcanic-Like Viral Factories with DIC and DAPI staining(http://www.ncbi.nlm.nih.gov/pmc/articles/PMC1828621/bin/pone.0000328.g005.jpg]] | ||
<br>Acanthamoeba polyphaga Mimivirus is one of the most well studied of the Nucleocytoplasmic Large DNA Viruses (NCLDVs), and therefore serves as a good focus when understanding megaviral replicative cycles. To begin replication Mimivirus first must enter a host, which is usually an Amoeba, although some evidence suggests that Mimivirus has had interaction with coral species(Claverie, 2009). After entering the host, Mimivirus must undergo a 4 hour eclipse phase, this relatively inactive period happens before any replication is initiated, then brand new Mimivirus particles are produced in the cell cytoplasm accumulating until the eventual lysis of the cell and they are released. Mimivirus first enters the amoeba cell by phagocytosis, meaning that amoeba become infected by way of their own feeding processes. Specifically, the Mimivirus membrane fuses with the cell vacuole membrane and releases what most reasonably is the viral DNA into the cell cytoplasm. Once this DNA enters the nucleus, overtime a complex forms in the amoeba that accumulates mass known as the virus factory(VF). This is where DNA encapsidation occurs. The capsid protein L425 is a scaffolding protein that encapsulates the highly accumulated virions in the cytoplasm (Abrahao, 2014) This sequence was elucidated through interpretation and comparison of Transmission Electron Microscopy(TEM) slides(Figure 6.) and is still a topic of active research. Through Difference Interference Contrast(DIC) microscopy, researchers were able to topographically visualize 10um tall volcano like features of the virus factory, With DAPI staining we can see that these volcano like structures are a focal point for newly synthesized DNA(Figure 7.).<br> | <br>Acanthamoeba polyphaga Mimivirus is one of the most well studied of the Nucleocytoplasmic Large DNA Viruses (NCLDVs), and therefore serves as a good focus when understanding megaviral replicative cycles. To begin replication Mimivirus first must enter a host, which is usually an Amoeba, although some evidence suggests that Mimivirus has had interaction with coral species(Claverie, 2009). After entering the host, Mimivirus must undergo a 4 hour eclipse phase, this relatively inactive period happens before any replication is initiated, then brand new Mimivirus particles are produced in the cell cytoplasm accumulating until the eventual lysis of the cell and they are released. Mimivirus first enters the amoeba cell by phagocytosis, meaning that amoeba become infected by way of their own feeding processes. Specifically, the Mimivirus membrane fuses with the cell vacuole membrane and releases what most reasonably is the viral DNA into the cell cytoplasm. Once this DNA enters the nucleus, overtime a complex forms in the amoeba that accumulates mass known as the virus factory(VF). This is where DNA encapsidation occurs. The capsid protein L425 is a scaffolding protein that encapsulates the highly accumulated virions in the cytoplasm (Abrahao, 2014) This sequence was elucidated through interpretation and comparison of Transmission Electron Microscopy(TEM) slides(Figure 6.) and is still a topic of active research. Through Difference Interference Contrast(DIC) microscopy, researchers were able to topographically visualize 10um tall volcano like features of the virus factory, With DAPI staining we can see that these volcano like structures are a focal point for newly synthesized DNA(Figure 7.).<br> | ||
==Megavirus Gene Products== | ==Megavirus Gene Products== |
Revision as of 23:21, 27 April 2016
By Adama Berndt
Introduction
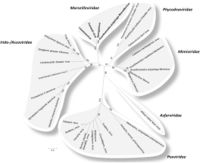
The evolutionary history of viruses is an unclear and frequent topic of speculation. Megaviruses are especially unique, as their size and structure are reminiscent of bacterial cells, but their function is similar to that of a classical virus(Abrahao, 2014). Although knowledge of large viruses dates back to the 1970’s, interest in megaviruses expanded during 2003 with the sequencing of Mimivirus; now one of the most well characterized Nucleocytoplasmic Large DNA Viruses(NCLDVs)(Colson, 2012). There are 6 official recognized classes of NCLDVs: Poxviridae, Asfarviridae, Iridoviridae, Ascoviridae, Phycoviridae, Mimiviridae(Colson, 2013; Fig 2.). In addition to these classes Megaviridae is proposed as a seventh class(Yutin and Koonin, 2012). One of the most remarkable recurring traits of these microbes is their large genome size, which at approximately one million base pairs is bigger than some bacterial genomes. These base pairs code for over a thousand genes and this genetic material is surrounded by pseudo-icosahedral protein capsids, similar to a Herpes virus(Legendre, 2012)(Fig. 1.). The taxonomy of viruses as DNA containing microbes has long been considered from many angles. Of special consideration is the status of NCLDVs as living or non-living organisms. From an evolutionary perspective, there are two major sides to this debate, the “virus-early” hypothesis the “virus-late’ hypothesis. The virus-early stance argues that viruses originated before modern cells and the virus-late stance speculates that viruses are the result of a reductive evolution, characterized by an increased benefit from reliance on host metabolism, which propelled the loss of primary cellular machinery. The other potential mechanism of the virus-late camp imagines the escape of proteins and genetic information from cells, that coupled to form viruses(Moreira, 2008). If viruses were formed spontaneously from released cell genetic materials and proteins, me might expect to see some formation of new rudimentary viruses today. The genetic information obtained from Mimivirus has provided extra fuel for speculation in this debate, specifically that Mimivirus contains the first evidence of a virus containing genes for transcription and translation(Raoult, 2007). The full phylogenetic analysis conducted by Raoult and colleagues found several Eukaryotic protein homologues in Mimivirus, and support for Mimivirus as a sister group to Eukaryotes and therefore a new branch of life that would include, Eukaryotes, Prokaryotes, Archaea and Megavirales.

Other examples:
Bold
Italic
Subscript: H2O
Superscript: Fe3+
Introduce the topic of your paper. What is your research question? What experiments have addressed your question? Applications for medicine and/or environment?
A citation code consists of a hyperlinked reference within "ref" begin and end codes.
[1]
The Evolution of Nucleocytoplasmic Large DNA Viruses
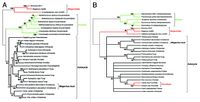
One of the most fascinating aspects of NCLDVs, is how they change the debate on viruses as life or not. NCLDVs share genes with all of the primary living domains; Archaea, Bacteria and Eukarya. It is especially integral that these genes are involved with the something as essential to life as DNA storage and processing(Abrahao, 2014). It begs the question how can these viruses contain so many genes required for life, and still not be considered life? In addition, it has recently been demonstrated that that Mimivirus contains its own glycosylation that leads to a 4-amino-4 6-dideoxy-D-glucose, called viosamine. It is also possible that this pathway could have evolved and changed to become similar eukaryotic glycosylation pathways. The presence of this sugar is observed even without the presence of regular amoeba host, suggesting the potential for autonomous, or semi-autonomous growth of Mimivirus(Piacente,2011). Environmentally the role of aquatic NCLDVs has also been imagined. With the suggestion being that the role of Mimiviruses perhaps was to lyse prokaryotes or eukaryotes and release carbon containing compounds, or other nutrients to increase the presence of microbes or any other systems or animals that might depend on this cell lysis. When it comes to considering virus-early or virus-late hypotheses, unfortunately there is not a clear cut answer in differing phylogenetic trees. Analysis of highly conserved genes suggest that both hypotheses are possible, Eukaryotes may share an ancestor for all NCLDVs, until this ancestor eventually diverged from eukaryotic life. It is also possible that Eukaryotes were already a distinguished group and played host to the ancestor of modern day NCLDVs, until their differentiation was mediated by horizontal virus transfer(Yutin and Koonin, 2010).
Include some current research, with at least one figure showing data.
Every point of information REQUIRES CITATION using the citation tool shown above.
Support For Megavirales as a Fourth Domain
The classification of NCLDVs as a defining phylogenetic group, or the establishment of Megavirales as a proposed new order is one that does not go unchallenged in the face of certain objections to phylogenies created from viral DNA. This is for two main reasons: Viruses evolve at an extremely rapid rate meaning that consistency will be difficult to maintain from one genetic analysis to the next, within a small set of constantly changing genes. The second reason is that viruses are liable to engage regularly in horizontal gene transfer with the host muddying up clear relationships between species or patterns of descent. However, in the case of NCLDVs these objections are less applicable. These double-stranded DNA viruses do not evolve at a rate comparable to smaller viruses with homologs that are significantly conserved, therefore we might expect the significant difference between NCLDVs to be a result of divergence that has occurred over a significant amount of time, suggesting a common ancestor for NCLDVs. The idea that horizontal gene transfer occurs regularly is also challenged in Megaviruses, due to their ability to rely completely on the cellular processes of the host. Therefore, there is not much evolutionary incentive for them to retain host genomes in NCLDV populations, in fact the pressure would suggest more static gene populations, or further reductive evolution, barring some unforeseen environmental shift. There is also not genetic evidence for clear-cut examples of gene transfer that has occurred between Eukaryotic hosts and their associated NCLDVs. For example, the open reading frames for non-homologous genes between Megavirus and MImivirus are 80% different, much higher than would be expected in the case of horizontal gene transfer. However, certain sets of genes (described further in gene product section) are sufficiently conserved in order to facilitate and form an adequate phylogeny (Legendre, 2012). To build a phylogeny, the Legendre paper used two specific genes that had excellent conservation in NCLDV’s and that were necessary for DNA replication. The first being a ribonucleoside diphosphate reductase sub-unit and a DNA replication clamp loader called factor C. This analysis illustrates that these genes show clear distinction from Archaea and Eukaryotes, showing little, or no overlapping affinities between these orders. Said another way, Megavirales as a proposed order, appears just as separate from Eukaryotes as Archaea are. Also up for speculation is whether or not Megavirales are at the root of the Eukaryotic domain as in Figure 2A, or are not evolutionary pre-cursors to Eukaryotes as in Fig. 2B.
However, phylogeny based on these two gene regions as in figure 3. are not necessarily the only kind a researcher could choose to build. In fact other work using DNA ligase as the primary gene to build phylogeny, has not seen such a clear cut relationship(Yutin and Koonin, 2012). When using DNA ligase Yutin and Koonin found that a presence-absence analysis of a particular kind of DNA ligase showed clear progression and separtion of an ancestral and current form. However, when supplementing the very same analysis with other DNA ligase genes, the likely ancestor became the more recent ligase and the other ligase was projected as the ancestor. This shows that although there is obvious support for a common ancestor for NCLDVs, not all phylogenies will place genes chronologically in the same order. Some phylogenies are not even able to statistically separate or group the proposed NCLDVs with significance. It all depends on the genes you choose to analyze them and weighting certain genes as more or less important is extremely complicated and difficult(Yutin and Koonin, 2012). Ultimately, this work suggests the opposite conclusions of other papers, and hints at the possibility of independent acquisition of genes by NCLDVs from cellular hosts, as a way of explaining difficulties in consistently classifying these different large viruses. Support for Megavirales as a new life classification is also slightly contested by the fact that Poxviridae and Ascoviridae, are both NCLDVs that don’t feature icosahedral capsids, instead they have brick-shaped allantoid capsids. However, in Poxviridae organisms it is thought that this feature is a derived one, due to the fact that during morphogenesis icosahedral form is expressed as intermediate form, and the D13 protein is still transcribed and translated during this developmental stage(Colson, 2013).
Sequencing Methods
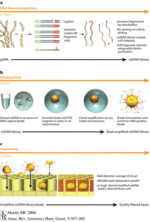
Roche-454 Pyrosequencing was one of the first methods to gain commercial use for rapid genetic sequencing and is in common use for obtaining the phylogeny analyses discussed earlier. Pyrosequencing is powered by the release of pyrophosphates at every nucleotide addition. This pyrophosphate release stimulates a cascade that results in the eventual light production by firefly enzyme luciferase. The measured light correlates to number of base pairs that have been constructed. (Mardis, 2008). First DNA is separated to form an ssDNA library by way of bonding to a multitude of agarose beads containing complementary oligonucleotide sequences. Then these beads are separated in mixtures of oil and water that isolate each bead in micelles with PCR reactants. This means that each bead ends up with millions of copies of a single strand of DNA. Each bead is then arranged and fixed in place by the wells of a picotiter plate. Agarose beads are then surrounded by enzyme-containing beads that are responsible for catalyzing associated reactions that facilitate nucleotide addition through the creation of downstream pathways. The isolated addition in all wells is read simultaneously by CCD cameras, as pure nucleotide solution is added. The emitted light allows the camera to tell which nucleotides are added and computer analysis may then align complementary strands to generate a whole sequence. This process affords 250 nucleotides per read, and the computer eliminates the presence of mixed beads with mixed initial ssDNA’s, or beads with DNA that is not affixed and is lacking the calibratory TCGA sequence. This system is not perfect however, due to the manner in which pyrosequencing is calibrated, reads of the same base over 6 times lead to a particular kind of error, although other errors are certainly possible, this is where other methods of sequencing can fill in the gaps.
The other method occasionally used in conjunction with Roche 454 pyrosequencing is a combination of Illumina and Sanger techniques(Arslan, 2011). For Illumina sequencing DNA is transected randomly and bound to Illumina adapter sequences at each end of DNA. These adapter sequences tether to oligonucleotide flow cells and complementary adapter strands bind together to form bridges that facilitate repeated amplification of DNA at flow cell clusters. Eventually strands are replicated in clusters of optimally a million identical DNA strands similar to the Roche agarose clusters and then they are separated. Then to finally sequence the data the addition of differential ACGT fluorescent nucleotides is imaged after each unique fluorescent base binds one at a time. Then the data is analyzed for read quality, and any bad reads are discarded. Random sequences of 25-35 base pairs are assessed for similarity and overlapped according to repeat sequences. This level of automation constructed in sequencing genetic information is takes up large amounts of data storage and requires a relatively powerful computer for the sequencing software algorithms, but the time and money saved as a result as revolutionized the research that can be done in genetic analysis. It is now possible to gain detailed and potentially fruitful information about the proteins coded for by the isolates of novel microbe genomes, such as those of NCLDVs.
Mimivirus Replication

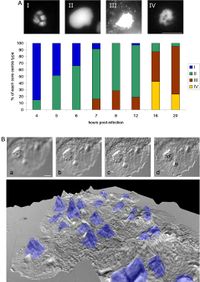
Acanthamoeba polyphaga Mimivirus is one of the most well studied of the Nucleocytoplasmic Large DNA Viruses (NCLDVs), and therefore serves as a good focus when understanding megaviral replicative cycles. To begin replication Mimivirus first must enter a host, which is usually an Amoeba, although some evidence suggests that Mimivirus has had interaction with coral species(Claverie, 2009). After entering the host, Mimivirus must undergo a 4 hour eclipse phase, this relatively inactive period happens before any replication is initiated, then brand new Mimivirus particles are produced in the cell cytoplasm accumulating until the eventual lysis of the cell and they are released. Mimivirus first enters the amoeba cell by phagocytosis, meaning that amoeba become infected by way of their own feeding processes. Specifically, the Mimivirus membrane fuses with the cell vacuole membrane and releases what most reasonably is the viral DNA into the cell cytoplasm. Once this DNA enters the nucleus, overtime a complex forms in the amoeba that accumulates mass known as the virus factory(VF). This is where DNA encapsidation occurs. The capsid protein L425 is a scaffolding protein that encapsulates the highly accumulated virions in the cytoplasm (Abrahao, 2014) This sequence was elucidated through interpretation and comparison of Transmission Electron Microscopy(TEM) slides(Figure 6.) and is still a topic of active research. Through Difference Interference Contrast(DIC) microscopy, researchers were able to topographically visualize 10um tall volcano like features of the virus factory, With DAPI staining we can see that these volcano like structures are a focal point for newly synthesized DNA(Figure 7.).
Megavirus Gene Products
All NCLDVs have 5 major defining genes in common. Poxvirus D13 codes for the primary capsid protein, D5 specifies a helicase-primase for the initiation of DNA replication. NCLDVs also share a DNA polymerase elongation sub-unit family protein, an A32 ATPase encoding region and an A2L gene specifying a viral late transcription factor. This suite of genes help to characterize NCLDVs in addition to their large size and a set of 50 other genes that are not common between NCLDVs, but together help to form a suite in a proposed ancestor for all the NCLDVs. These 50 genes also clearly separate NCLDVs phylogenetically from other large viruses which interact pathogenically with Eukaryotic organisms like herpesvirus, in addition to other large prokaryotic and archaea infecting large viruses (Colson, 2013). NCLDVs also share double-stranded DNA and similar replicative cycles that create viral factories in the cytoplasm.
Megavirus Human Interaction
Although Mimivirus and Megavirus chilensis are known to interact with amoeba and not directly with human hosts, other NCLDVs like the small pox virus have significant human interaction. Small pox is classified as a member of the Poxviridae family, which contains a diverse set of pathogenic viruses that parasitize other animals, such as rabbit myxoma virus and vaccina virus. Poxviridae members also have parasitic interactions with unicellular eukaryotes(Koonin, 2010). Even though Mimivirus doesn’t have a clear pathogenic interaction with humans, unsurprisingly the discovery of Mimivirus was a direct result of research aimed at addressing pneumonia infections associated with the water-tower amoeba that Mimivirus were using as hosts(Abrahao, 2014).
Megavirus Chilensis
Megavirus Chilensis was recently discovered in 2011 contained in Chilean coastal amoeba. It is noteworthy as a distant relative to the NCLDV Mimivirus and currently stands as the largest known megavirus at approximately 700nm in diameter including icosahedral capsid and virus “hairs”(Arslan, 2011). Even though the structures of Mimivirus and Chilensis are remarkably similar, they differ significantly in terms of genetics, with only 50% of residues being conserved on average between any given Mimivirus and Chilensis viral particles. In addition to this Chilensis has 594 orthologs with Mimivirus out of the roughly 1000 gene products each NCLDV type makes. This puts Chilensis and Mimivirus at the borderline of maximum difference usually considered within a bacterial classification (i.e. firmicutes.). Chilensis is also unique for its three extra amino-acyl tRNA synthetases, relative to Mimivirus(Arslan, 2011).
Virophages

NCLDVs such as Mimiviridae are remarkable discoveries in their own right, but their finding also led to the awareness of the equally remarkable virophages. Virophages are tiny satellite viruses. The first characterized was the sputnik virus, a viral particle only 50nm in diameter and with capsids of icosahedral form. The DNA is similarly small counting in at 18kb, which gives them a small theorized coding capacity of only 21 proteins. A few of these proteins are completely novel in having no homologous nature with other viral genomes. The sputnik virus interacts directly with Mimivirus viral factories inhibiting the NCLDV’s normal replication in amoeba and pathogenicity by 70% and using the viral factories for it’s own reproduction(Fig. 8). Current research hints at the possibility of virophages acting as a part of a NCLDV’s mobile genetic elements, meaning that virophage virions become integrated thoroughly in the genome. This is the case for a different virophage called Lentile virus, which also has transpovirons, that can recombinate with other viruses such as sputnik2. Therefore, NCLDVs create and facilitate the exchange of genes between these virophages, and this interaction could be beneficial in better understanding the complex genomes of NCLDVs(Abrahao, 2014).
Conclusion
References
- ↑ [http://www.karger.com/Article/Fulltext/336562 Colson, Phillipe. Lamballerie, Xavier de. Fournous, Ghislain. Raoult, Didier. Reclassification of Giant Viruses Composing a Fourth Domain of Life in the New Order Megavirales ." Intervirology 2012;55:321-332. DOI: 10.1159]
Authored for BIOL 238 Microbiology, taught by Joan Slonczewski, 2016, Kenyon College.