Magnetotactic bacteria and their application: Difference between revisions
No edit summary |
No edit summary |
||
Line 27: | Line 27: | ||
==Physiology and Ecology== | ==Physiology and Ecology== | ||
MTB usually inhabit sediments and water columns with vertical chemical stratification. Like many, bacteria, MTB’s main mode of taxis is through their helical flagella. Magnetotaxis causes these bacteria to orient themselves northward along geographic lines while in the northern hemisphere, and southward while in the southern. Interestingly enough, MTB are observed to swim both directions while along the equator. As a general rule, MTB are anaerobes, microaerophiles or facultatively anaerobic microaerophiles. Because of this, they are most likely to found at the the borders of oxic-anoxic regions as well as the anoxic regions of their habitat. In terms of a more physical placement within their habitat, MTB are most likely to be found at the water-sediment interface or thereabouts. | MTB usually inhabit sediments and water columns with vertical chemical stratification. Like many, bacteria, MTB’s main mode of taxis is through their helical flagella. Magnetotaxis causes these bacteria to orient themselves northward along geographic lines while in the northern hemisphere, and southward while in the southern. Interestingly enough, MTB are observed to swim both directions while along the equator. As a general rule, MTB are anaerobes, microaerophiles or facultatively anaerobic microaerophiles. Because of this, they are most likely to found at the the borders of oxic-anoxic regions as well as the anoxic regions of their habitat. In terms of a more physical placement within their habitat, MTB are most likely to be found at the water-sediment interface or thereabouts. | ||
A number of morphological types can be found within the MTB group including cocci, spirilla, vibrios and multicellular forms. MTB biomineralized two groups of metals: iron oxides and iron sulfides. Those species that produce iron sulfides biomineralized greigite (Fe3S4), while those that produce iron oxides biomineralized magnetite (Fe3O4). Additionally, species have been found that produce both and have magnetosomes that contain both magnetite and greigite. | A number of morphological types can be found within the MTB group including cocci, spirilla, vibrios and multicellular forms. MTB biomineralized two groups of metals: iron oxides and iron sulfides. Those species that produce iron sulfides biomineralized greigite (Fe3S4), while those that produce iron oxides biomineralized magnetite (Fe3O4). Additionally, species have been found that produce both and have magnetosomes that contain both magnetite and greigite. | ||
Geographic distribution of MTB’s are as widely varied as their morphology. As of today, only oxide-producers have been found in freshwater environments, which range from North America to Asia. MTB are even found within more extreme environments, such as lake Mono in California, an alkaline and saline in which the low solubility of iron makes the survival of MTB very difficult. Additionally, MTB are commonly found within the anoxic layers of marine environments, usually near the sedimentary layer. | Geographic distribution of MTB’s are as widely varied as their morphology. As of today, only oxide-producers have been found in freshwater environments, which range from North America to Asia. MTB are even found within more extreme environments, such as lake Mono in California, an alkaline and saline in which the low solubility of iron makes the survival of MTB very difficult. Additionally, MTB are commonly found within the anoxic layers of marine environments, usually near the sedimentary layer. | ||
== | ==The Magnetosome== | ||
The magnetosome, the most important and defining part of the MTB, is an intracellular, membrane-surrounded magnetic iron inorganic crystal. Since its discovery, researchers have recognized it as a true prokaryotic organelle because of its complexity that rivals that of eukaryotic organelles. Despite its universal presence within MTB, the size, composition and morphology of the magnetosome varies between species. Usually, the size of the magnetosome ranges from 25mm-125mm, although 250nm magnetosomes have been observed in uncultured freshwater cocci. At normal temperatures, magnetosomes are permanently magnetic and form stable single-magnetic domains (meaning that they are uniformly magnetic). However, beyond normal temperatures and size ranges, magnetosomes are either unreliably magnetic or form multiple fields at smaller and larger than average temperatures, respectively. In terms of morphology, a few different forms of magnetosomes have been observed across species by various forms of electron microscopy. These include, rectangular, bullet-shaped, octahedral and elongated prismatic morphologies. In most strains of MTB, the magnetosomes are fixed in several chains that are in turn attached to the cell in order to limit magnetostatic energy. The most commonly used minerals in the magnetosomes are, as mentioned previously, magnetite or greigite. However, many other iron sulfides have also been found within sulfide producing MTBS, such as cubic FeS and tetragonal FeS. The membrane that surrounds the crystal acts as a gate for pH, compositional and redox differentiation between the cellular environment and the vesicle. Its composition is thought be made up primarily of lipids, proteins, fatty acids, glycolipids, phospholipids and sulfolipids. | |||
The current model for Magnetosome formation consists of a few different processes:: vesicle formation, extracellular iron intake, iron transport into the magnetosome, and mineralization—although this knowledge mainly comes from the Magnetospirillum species, which is more readily culturable than other MTB. While it is known through direct observation (as well as common sense) that the later three steps are ordered temporally, it is unknown if vesicle formation comes before the uptake of iron or not. The most recent proposed model by Arakaki et al. (2008) lists three discrete steps. The first step consists of the creation of magnetosome membrane invaginations out of the cytoplasmic membrane (primed by GTPase), as well as the lining up of these vedicles along cytoskeletal filaments in order to form a chain.. Second, iron is transported into the cell. This transport is strictly regulated by an oxidation-reduction system because of the potentialy dangerous effects of having too much intracellular iron. In the third and final step, magnetosome proteins regulate morphology and trigger magnetite crystal nucleation. | |||
==Section 3== | ==Section 3== |
Revision as of 03:28, 29 April 2016
Section

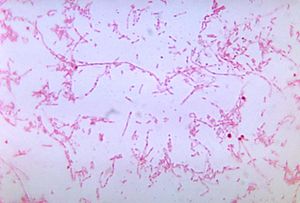
By Grant Miner
At right is a sample image insertion. It works for any image uploaded anywhere to MicrobeWiki.
The insertion code consists of:
Double brackets: [[
Filename: PHIL_1181_lores.jpg
Thumbnail status: |thumb|
Pixel size: |400px|
Placement on page: |right|
Legend/credit: Electron micrograph of the Ebola Zaire virus. This was the first photo ever taken of the virus, on 10/13/1976. By Dr. F.A. Murphy, now at U.C. Davis, then at the CDC.
Closed double brackets: ]]
Other examples:
Bold
Italic
Subscript: H2O
Superscript: Fe3+

Overview
Magnetotactic bacteria (MTB) are a polyphyletic group of Gram-negative prokaryotes. Members of this group are characterized by their ability to align themselves and swim along a magnetic field. They owe this ability to the magnetosome, a specific intracellular structure that contains crystals of of magnetic metals (usually magnetite or greigite) bounded by a membrane. They were discovered in 1975 by Richard Blakemore. In his paper “Magnetotactic Bacteria” (Blakemore coined the name), which he observed bacteria migrating along geomagnetic lines and, upon investigation, the magnetosome. This discovery impacted the scientific communities across many disciplines, including geology, mineralogy, chemistry, biochemistry, physics, oceanography, and, of course, microbiology. Because all species of MTB require microaerobic conditions, few species have been cultivated in pure condition. Because of this, they are relatively difficult to use in biotechnology. Nevertheless, researchers have succeeded in making headway in utilizing these strange organisms.
Physiology and Ecology
MTB usually inhabit sediments and water columns with vertical chemical stratification. Like many, bacteria, MTB’s main mode of taxis is through their helical flagella. Magnetotaxis causes these bacteria to orient themselves northward along geographic lines while in the northern hemisphere, and southward while in the southern. Interestingly enough, MTB are observed to swim both directions while along the equator. As a general rule, MTB are anaerobes, microaerophiles or facultatively anaerobic microaerophiles. Because of this, they are most likely to found at the the borders of oxic-anoxic regions as well as the anoxic regions of their habitat. In terms of a more physical placement within their habitat, MTB are most likely to be found at the water-sediment interface or thereabouts.
A number of morphological types can be found within the MTB group including cocci, spirilla, vibrios and multicellular forms. MTB biomineralized two groups of metals: iron oxides and iron sulfides. Those species that produce iron sulfides biomineralized greigite (Fe3S4), while those that produce iron oxides biomineralized magnetite (Fe3O4). Additionally, species have been found that produce both and have magnetosomes that contain both magnetite and greigite.
Geographic distribution of MTB’s are as widely varied as their morphology. As of today, only oxide-producers have been found in freshwater environments, which range from North America to Asia. MTB are even found within more extreme environments, such as lake Mono in California, an alkaline and saline in which the low solubility of iron makes the survival of MTB very difficult. Additionally, MTB are commonly found within the anoxic layers of marine environments, usually near the sedimentary layer.
The Magnetosome
The magnetosome, the most important and defining part of the MTB, is an intracellular, membrane-surrounded magnetic iron inorganic crystal. Since its discovery, researchers have recognized it as a true prokaryotic organelle because of its complexity that rivals that of eukaryotic organelles. Despite its universal presence within MTB, the size, composition and morphology of the magnetosome varies between species. Usually, the size of the magnetosome ranges from 25mm-125mm, although 250nm magnetosomes have been observed in uncultured freshwater cocci. At normal temperatures, magnetosomes are permanently magnetic and form stable single-magnetic domains (meaning that they are uniformly magnetic). However, beyond normal temperatures and size ranges, magnetosomes are either unreliably magnetic or form multiple fields at smaller and larger than average temperatures, respectively. In terms of morphology, a few different forms of magnetosomes have been observed across species by various forms of electron microscopy. These include, rectangular, bullet-shaped, octahedral and elongated prismatic morphologies. In most strains of MTB, the magnetosomes are fixed in several chains that are in turn attached to the cell in order to limit magnetostatic energy. The most commonly used minerals in the magnetosomes are, as mentioned previously, magnetite or greigite. However, many other iron sulfides have also been found within sulfide producing MTBS, such as cubic FeS and tetragonal FeS. The membrane that surrounds the crystal acts as a gate for pH, compositional and redox differentiation between the cellular environment and the vesicle. Its composition is thought be made up primarily of lipids, proteins, fatty acids, glycolipids, phospholipids and sulfolipids.
The current model for Magnetosome formation consists of a few different processes:: vesicle formation, extracellular iron intake, iron transport into the magnetosome, and mineralization—although this knowledge mainly comes from the Magnetospirillum species, which is more readily culturable than other MTB. While it is known through direct observation (as well as common sense) that the later three steps are ordered temporally, it is unknown if vesicle formation comes before the uptake of iron or not. The most recent proposed model by Arakaki et al. (2008) lists three discrete steps. The first step consists of the creation of magnetosome membrane invaginations out of the cytoplasmic membrane (primed by GTPase), as well as the lining up of these vedicles along cytoskeletal filaments in order to form a chain.. Second, iron is transported into the cell. This transport is strictly regulated by an oxidation-reduction system because of the potentialy dangerous effects of having too much intracellular iron. In the third and final step, magnetosome proteins regulate morphology and trigger magnetite crystal nucleation.
Section 3
Include some current research, with at least one figure showing data.
Section 4
Conclusion
References
Authored for BIOL 238 Microbiology, taught by Joan Slonczewski, 2016, Kenyon College.