The Role of Methanogens in Waste Water Treatment: Difference between revisions
Mooradianm (talk | contribs) |
Mooradianm (talk | contribs) |
||
Line 33: | Line 33: | ||
==Conclusion== | ==Conclusion== | ||
<br> | <br>Methanogens play a vital role in the treatment of wastewaters across the globe. Through methanogenesis, methanogens utilize organic pollutants as sources of energy, producing methane gas as a byproduct. The process of methanogenesis occurs in anaerobic environments, making it an ideal process for aquatic and wetland environments. These microbes are extremely effective at breaking down organic pollutants and also provide a cost effective method of wastewater treatment. However, the use of methanogens also has a potentially negative environmental impact. Methanogens produce large quanities of methane as a byproduct, which contributes to the overall greenhouse effect. Current research is being done in order to quantify the full effects of these emissions and determine if the use of methanogens in wastewater treatment is sustainable for the long term. <br> | ||
==References== | ==References== |
Revision as of 01:29, 23 April 2014
Introduction
by Melissa Mooradian
As one of the most diverse groups of Archaea known to date, the Methanogens represent the euryarchaeota order which gains energy via methane production. The methanogens utilize the metabolic process of methanogenesis, which is the methane production pathway that converts carbon dioxide and other bacterial waste products into methane. Methanogens have become the focus of recent literature due to their large contribution to global methane emissions as well as their role in wastewater treatment. Methane is a potent greenhouse gas that has a global warming potential of 25 in a 100 year time frame and is estimated to account for 20% of the total radiative forcing from all greenhouse gases (Wang et al., 2011). The recent increase in methane emissions, from natural systems such as wetlands as well as landfills and agricultural cattle, has made the understanding of methanogens crucial to reducing the effects of climate change. Although methane emissions have negative environmental impacts, the use of methanogens can be crucial in reducing wastewater and the pollution of water systems across the world. Methanogens help break down organic material that would otherwise pollute water sources and lead to environmental degradation. The construction of wetland systems in developing countries has been adopted as a low cost and highly effective method of reducing nutrient concentrations and degrading organic compounds in agricultural as well as urban wastewaters (Johansson, et al., 2004). The balance of these two impacts is vitally important for the future.
Methanogens

The methanogens are a group of Archaea within the euryachaeota order that perform methanogenesis (Figure 1). Methanogens are among the most diverse groups of microbes and differ significantly in their environmental conditions and cell structure. However, all methanogens require anaerobic environments, as the presence of oxygen blocks the process of methanogenesis from occurring (Slonczewski and Foster, 2014). There are over 50 described species of methanogens, which fall into five distinct orders (Figure 2). Methanogenesis can occur via six major reactions, each using different substrates. These different pathways help categorize the five orders of methanogenesis as well as determine the different environments each species is found in. The first order, the methanobacteriales, are rod shaped methanogens that utilize hydrogen and carbon dioxide in methanogenesis (Slonczewski and Foster, 2014). They are found at higher pH, ranging from 6 to 9, and a variety of temperatures. The second order, the methanococcales, are cocci which contain flagella and also utilize hydrogen and carbon dioxide (Slonczewski and Foster, 2014). The third order, the methanomicrobiales, utilizes acetate for methanogenesis (Slonczewski and Foster, 2014). This group is found at lower temperatures and can the cell shape varies from cocci to spirilla. The fourth order is the methanopyrales, a group of rod shaped methanogens that utilize carbon dioxide and hydrogen (Slonczewski and Foster, 2014). This group is found at extremely high temperatures. The final group is the methanosarcinales. This group is found in mostly low temperatures but has varying cell shape and methanogenesis substrates. They range from cocci to filamentous rods and metabolize almost every substrate used for methanogenesis (Slonczewski and Foster, 2014). The substrate used for methanogenesis also determines whether the species is a autotroph or a heterotroph. Those reducing carbon dioxide are considered autotrophs where as those utilizing other substrates are considered heterotrophs (Slonczewski and Foster, 2014).
Although methanogens are highly diverse, they all contain certain key features. One of those features is the presence of a rigid cell wall (Slonczewski and Foster, 2014). This helps the cell maintain its distinctive shape, even in extreme environments. A second trait is their reliance on syntrophy. Syntrophy is the close association of an archaea with a bacterial partner in order to be provided with key substrates. Almost all methanogens participate in this practice in order to obtain the substrates used in methanogenesis. All methanogens must also be cultured in anaerobic chambers, due to the inability for methanogenesis to occur in the presence of oxygen. Finally, many methanogens are filamentous. This trait is especially useful in wastewater treatment because the bacterial partners necessary to provide the appropriate substrates get trapped within the filaments (Slonczewski and Foster, 2014).
Methanogens are also found in a variety of different environments. The three most common habitats for microbes are soils, animal digestive tracts, and marine floor sediments. The soils of wetland systems are the most common and have received the most attention in the literature due to the methane emissions from wetland systems as well as their role as carbon sinks. Soil environments containing methanogens must be anaerobic, since methanogenesis cannot occur in the presence of oxygen. Due to this, wetland soils, which are usually anoxic, have some of the largest producers of methane where as drained soils often serve as methane sinks (Uggetti et al., 2012). The activity of methanogens and overall methane production from wetlands is therefore regulated by oxygen levels, water level, plant communities, and temperature (Uggetti et al., 2012). These varying factors mean than wetland systems can act as either methane sinks or sources depending on the rate of methane production and ability of the soils to sequester carbon. Landfills are also an important environment for methanogens. Landfills are rich in organic wastes, causing methane to build up within the system. Methane from these landfills must be pumped out in order to avoid explosions and can be collected and used for electricity (Slonczewski and Foster, 2014). Methanogens are also extremely common in the digestive tracts of animals, including termites, cattle, and humans. Methane emissions from agricultural cattle have increased significantly as industrial agriculture has taken over much of the country’s farming practices, which has contributed greatly to the overall greenhouse effect of methane. Methanogens in the human digestive tract account for 10% of all gut anaerobes and help increase the efficiency of digestion (Slonczewski and Foster, 2014). The final environment common for methanogens is in marine floor sediments. Methanogens at or beneath the seafloor form methane gas hydrates, which are methane molecules surrounded by water molecule cages (Slonczewski and Foster, 2014). These methane gas hydrates are a potential source of natural gas.
Methanogenesis
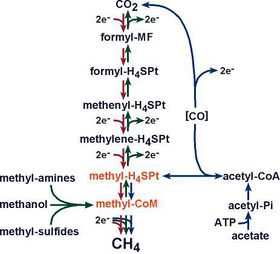
Methanogenesis is the methane production pathway methanogens use to obtain energy (Figure 3). This process involves the fermentation of carbon dioxide or other organic compounds with methane gas as the end product. Methanogenesis has six major pathways, each converting a different substrate into methane gas. The most common pathway converts carbon dioxide into methane gas through the reduction of hydrogen (Slonczewski and Foster, 2014). The other five pathways convert organic acids, produced through bacterial fermentation, into methane gas through the use of various methanogen specific cofactors. The six major substrates used are carbon dioxide, formic acid, acetic acid, methanol, methylamine, and dimethyl sulfate (Slonczewski and Foster, 2014). Each of these pathways is utilized depending on the species of methanogens present and the substrates available in the environment. Methane is therefore a byproduct of this anaerobic decomposition process that mains to break down organic acids and produce energy for the microbes present in the environment (Wang et al., 2011).
Although the role of methanogens and methane production has been extensively studied, the exact process and pathway of methanogenesis is not well described in most literature. It is often simply described as the conversion of organic acids or carbon dioxide into methane gas (Toprak, 1995). The true process of methanogenesis is much more complex and requires specific substrates and cofactors in order to occur. The two most common methanogenesis substrates are carbon dioxide and acetate. In the carbon dioxide pathway, CO2 is converted into methane and water via the passing of carbon down a series of cofactors. Carbon dioxide is fixed using hydrogen into methanofuran. The carbon is then passed down three cofactors, H4MPT, F420, and HS-CoM, until the carbon reaches HS-CoB, which serves as the terminal electron acceptor (Slonczewski and Foster, 2014). This process depends on the concentration of hydrogen ions as well as the sodium potential in order to donate electrons from CO2 and drive the ATP synthase that ultimately produces energy for the methanogens (Slonczewski and Foster, 2014). Due to this, methanogens, unlike many other microbes, require sodium ions for growth. Methanogenesis using the substrate acetate is not as well understood but is important in wastewater treatment. Wastewater contains high levels of organic acids, making acetate a more common substrate for methanogenesis than carbon dioxide. Methanogenesis from acetate requires the coupling of H2 concentration and a sodium potential in order to occur and uses the cofactors HS-CoM and HS-CoB in order to produce methane (Slonczewski and Foster, 2014). Unlike carbon dioxide methanogenesis, which produces water as a waste product, acetate methanogenesis produces a molecule called CoM-S-S-CoB, which is a converged form of the two carbons initially entered into the system (Slonczewski and Foster, 2014).
Methanogenesis in wastewater systems occurs as described above with an added step at the beginning of the process. This step, which occurs in two parts, is the conversion of waste products, organic compounds, into substrates able to be used by methanogens. First, aerobic respiration or fermentation by bacteria occurs to hydrolyze waste products into organic products such as aldehydes, alcohols, or, most commonly, organic acids (Toprak, 1995). After, hydrogen-producing bacteria break these products into hydrogen, carbon dioxide, and acetate, which can then be used in methanogenesis (Toprak, 1995). This two part process has also been described as the breakdown of raw waste into fatty acids via primary producers such as acidogens (Tabatabaei et al., 2010). Finally, after methanogenesis is completed, methane is relseased from the wastewater through surface diffusion or mechanical aeration (Wang et al., 2011). This process means that methanogens require syntrophy with bacterial partners in order to perform methanogenesis in wastewater. These bacterial partners are necessary for producing the substrates able to be utilized by methanogens.
Uses in Wastewater Treatment
Due to the ability of methanogens to break down organic acids efficiently, anaerobic fermentation has been used widely in wastewater treatment. It is a highly cost effective method and is therefore used in many developing countries. Although many studies have focused on the potential negative consequences of increasing the amount of methane entering the atmosphere, it has also been suggested that the methane produced in these wastewater treatment plants can be harvested and used as energy sources (Tabatabaei et al., 2010). Due to the wide diversity of methanogens, they have the ability to be used in many different environments and with various types of wastewater. Many wastewater treatment systems have also been designed in order to best degrade toxic compounds in the water and minimize the amount of methane being emitted into the atmosphere. The main types of wastewater in which methanogens are used are agricultural and industrial wastewaters.
Up to this point, not much research has been done on the specific types of wastewater that utilize methanogenesis but it is known that the species present differ depending on the composition of the water (Tabatabaei et al., 2010). In waters containing high levels of suspended solids and soluble organic pollutants, Methanosaeta is the dominant genus. These waters often contain high levels of acetate, which Methanoseata metabolizes readily (Tabatabaei et al., 2010). However, Methanoseata is highly sensitive to ammonia and is therefore not found in many other wastewater environments. In wastewaters containing high levels of ammonia or volatile fatty acids, such as dairy waste, Methanosarcinaceae is the dominant genus. Methanosarcinaceae are also able to effectively metabolize acetate (Tabatabaei et al., 2010). Wastewaters high in milk proteins often contain the genus Methanococcus, however this genus is not commonly found in many other wastewater systems (Tabatabaei et al., 2010). Finally, the genus Methanobacteriaceae is dominant in waters with high levels of BOD, biochemical oxygen demand, and COD, chemical oxygen demand (Tabatabaei et a;., 2010). COD concentrations have been found to be one of the strongest inhibitors of methanogen activity. Anaerobic activity, as well as methane production, decreases as COD loading rates increase (Toprak, 1995). These waters often contain molecules, such as tannins, that can be toxic to many other methanogen groups. Overall, a diverse array of methanogens are utilized in the treatment of wastewaters depending on the chemical composition of the water and the type of pollutants present.
Due to the diverse types of wastewater and methanogen species used, a few different types of treatment systems have been put into place. These treatment systems utilize a series of anaerobic reactors in order to break down the organic pollutants and allow methanogenesis to occur. These reactors also often try to capture the methane produced from this process to be used as a source of electricity. The most common reactor is called the class A design, which includes the closed digester tanks (CDT) and continuously stirred tank reactor (CSTR) (Figure 4). Both of these tanks work by providing equal retention time of the mathenogens, solid waste, and liquid waste (Tabatabaei et al., 2010). These two reactors are also the lowest in cost and simplest to operate, making them the most commonly used. In the CDT reactor, the substrate enters into the bottom inlet where it is mixed with a centrifuge pump (Figure 4). This mixing process allows for direct contact of the methanogens with the substrate and also allows for the release of methane gas from the substrate (Tabatabaei et al., 2010). The produced gas is then flowed through the top outlet where it can be processed to make biogas and the treated water exits the reactor system. The CSTR reactor works in almost exactly the same way, using an agitator to mix the substrate instead. The second class of reactors, the class B reactors, have been found to be more efficient but are also higher in cost and operation time. This groups contains the CSTR and CDT reactors combined with a solid recycling system and the upflow anaerobic sludge blanket bioreactor (UASB) (Tabatabaei et al., 2010). The class B reactors are thought to be more efficient due to the higher retention time of the methanogens and solids. The class B CSTR and CDT reactors function in the same way as the class A reactors with a settling tank added on. This system recycles the biomass the reactor and also provides the reactor with nutrients and alkalines (Tabatabaei et al., 2010). The UASB functions most like the other class B reactors but with a higher concentration of acidogens and methanogens at the bottom of the reactor. This high concentration is referred to as the sludge blanket and is thought to increase the efficiency of the reactor (Tabatabaei et al., 2010). It has been found that the use of sludge increases methane emissions, which in turn means higher methanogen activity (Uggetti et al., 2012). It has also been found that anaerobic digestion in sludge account for three quarters of the methane emission from wastewater treatment plants (Daelman et al., 2012). Although increased methane emissions may be harmful for the environment, the increased activity of methanogens means that the reactors in these wastewater treatments are effectively breaking down organic pollutants and producing cleaner water. This also indicates the focusing on the efficiency of sludge tanks can help decrease the methane emitted to the atmosphere by capturing the gas before it exits the tank and using it for purposes such as electricity (Daelman et al., 2012).
A popular emerging treatment system is the A/A/O process, the anaerobic/anoxic/oxic process. This process has become popular in large-scale wastewater treatment plants particularly in China (Wang et al., 2011). This process is typically used to clean waters high in nitrogen and phosphorus. The A/A/O process occurs in three steps. First, the water flows into anaerobic tanks containing agitators (Wang et al., 2011). Similarly to the class A reactors, the agitators are used to keep the sediment suspended and allows for the methanogens to have access to the methanogenesis substrates. Next, the water flows into the anoxic tanks where denitrification and nitrogen removal occur (Wang et al., 2011). Due to the low oxygen and nitrite levels in the first two tanks, high levels of methanogenesis occur. The third and final step is the oxic tanks which contain aeration equipment (Wang et al., 2011). This is where nitrification as well as phosphate removal and BOD decomposition occurs. This process has elements that are similar to the class A and B reactors but is thought to be more efficient. Since methane has a low water solubility and production occurs at multiple steps in this process, emissions from this system occur rapidly (Wang et al., 2011). It has also been found that in this process, similar to the UASB reactor, methanogenesis in the sludge contributes to the majority of the methane emission (Figure 5) (Wang et al., 2011). It has also been found that dissolved oxygen, organic loading rates, and nitrite concentrations can affect methane emission from this system, and thus affect the activity of methanogens. As mentioned before, methanogenesis is an anaerobic process and is inhibited by high levels of dissolved oxygen. Although the presence of oxygen in some parts of the process can be beneficial in order to prevent the production of excessive methane, aeration can also dissolved methane to be stripped from the water and therefore increase emissions (Wang et al., 2011). The A/A/O process effectively decreases the impact that organic loading rates have on methane emissions. Usually increased organic loading rates lead to increases in methane emissions but the switch between anaerobic and oxic tanks in this process decreases that negative effect (Wang et al., 2011). Finally, this process also effectively decreases the impact of nitrite on the activity of methanogens. Methanogenesis is inhibited by high nitrite concentrations however this process maintain lows levels of nitrite and thus also for methanogenesis to occur (Wang et al., 2011). Overall these three processes, the class A reactors, class B reactors, and the A/A/O process effectively use methanogenesis to remove detrimental compounds from various wastewater systems.
Possible Environmental Impacts
The use of methanogens in wastewater treatment has various environmental impacts. On a positive note, this process is an extremely easy and cost effective way to decrease pollutants in water sources. Wastewater from industrial and agricultural sources has become a major cause of aquatic ecosystem degradation and losses in biodiversity. As nutrients and pollutants from these sources enter water systems, the water chemistry changes and these ecosystems become inhabitable for many aquatic organisms. This leads to widespread losses in biodiversity of plant as well as aquatic animal communities. Furthermore the pollution of water systems has large-scale consequences for the global human population. Shortages of freshwater are occurring around the globe and clean sources of drinking water are vitally important for sustaining our population. Due to these reasons, the use of methanogens in maintaining health aquatic systems is extremely important.
Although methanogens can help preserve the health of aquatic ecosystems, high levels of methanogenesis can increase methane emissions dramatically and lead to increase in global greenhouse gas emissions. Methane is a potent greenhouse gas that contributes to 20% of all radiative forcing. Due to this, many researchers believe that the use of methanogens should not be used in wastewater treatments. It has been argued that these emissions do more harm to the environment than the good done by the cleaning of wastewaters. Although these treatment plants do cause increases in methane emissions, many strategies to mitigate the effect of these emissions have been proposed. First, the collection of methane produced from this processes to be used as sources of electricity may become a viable option in the future. Also, as mentioned previously, environmental conditions can be altered in order to decrease the activity of methanogens while still allowing them to fix pollutants in the water. Future research in this area is necessary in order to determine the full environmental effects of using methanogens in wastewater treatment plants.
Conclusion
Methanogens play a vital role in the treatment of wastewaters across the globe. Through methanogenesis, methanogens utilize organic pollutants as sources of energy, producing methane gas as a byproduct. The process of methanogenesis occurs in anaerobic environments, making it an ideal process for aquatic and wetland environments. These microbes are extremely effective at breaking down organic pollutants and also provide a cost effective method of wastewater treatment. However, the use of methanogens also has a potentially negative environmental impact. Methanogens produce large quanities of methane as a byproduct, which contributes to the overall greenhouse effect. Current research is being done in order to quantify the full effects of these emissions and determine if the use of methanogens in wastewater treatment is sustainable for the long term.
References
[8][Slonczewski, J.L., and Foster, J.W. "Microbiology: An Evolving Science". W.W. Norton and Company. 2014. Third Edition.]
Edited by student of Joan Slonczewski for BIOL 238 Microbiology, 2009, Kenyon College.