Symbiotic Microorganismal Toxin Production in Pufferfish: Difference between revisions
No edit summary |
No edit summary |
||
Line 1: | Line 1: | ||
<!-- Do not edit this line-->{{Slonczewski}}<br>By [Richard Dennis]<br> | <!-- Do not edit this line-->{{Slonczewski}}<br>By [Richard Dennis]<br> | ||
Tetrodotoxin, one of the most toxic biologically produced compounds known to mankind, is well-known for being produced by tetraodontidae, a family of fish known to include pufferfish and blowfish. The toxin is highly effective, presenting an LD50 of 10.7 µg/kg via injection and 532 µg/kg via ingestion in mice<ref name="Lago">[http://www.mdpi.com/1660-3397/13/10/6384 Lago Jorge, Rodriguez Laura P., Blanco Lucia, Vieites Juan Manuel, and Cabado Ana G. 2015. “Tetrodotoxin, an Extremely Potent Marine Neurotoxin: Distribution, Toxicity, Origin, and Therapeutic Uses” <i>Marine Drugs</i>. <b>13</b>(10), 6384-6406, doi:10.3990/md13106384]</ref>, and species such as Takifubu rubripes utilize the toxin actively as a self-defense mechanism <ref name="Matsumoto">[http://www.sciencedirect.com/science/article/pii/S0041010114005984 Matsumoto Takuya, Kiriake Aya, Ishizaki Shoichiro, Watabe Shugo, and Nagashima Yuji. 2015. “Biliary excretion of tetrodotoxin in the cultured pufferfish Takifugu rubripes juvenile after intramuscular administration” <i>Toxicon</i> <b>93</b>: 98-102.]</ref>. The toxin is frequently described as “over a thousand times more toxic than cyanide” <ref name="Bane">[http://www.mdpi.com/2072-6651/6/2/693/htm Bane Vaishali, Lehane Mary, Dikshit Madhurima, O’Riordan Alan, and Furey Ambrose. 2014. “Tetrodotoxin: Chemistry, Toxicity, Source, Distribution, and Detection”. <i>Toxins</i> (Basel) <b>6</b>(2): 693-755.]</ref> as a descriptor of its toxicity. However, toxic species such as these which have previously been thought to be prime producers of tetrodotoxin have been shown to become significantly less toxic when grown in non-toxic laboratory settings, indicating that production of tetrodotoxin might not be a biological process endogenous to this group <ref name="Noguchi">[https://www.researchgate.net/publication/44612067_Toxicity_of_pufferfish_Takifugu_rubripes_cultured_in_netcages_at_sea_or_aquaria_on_land Noguchi Tamao, Arakawa Osamu, Takatani Omohiro. 2006. “Toxicity of pufferfish Takifugu rubripes cultured in netcages at sea or aquaria on land.” <i>Comparative Biochemistry and Physiology Part D: Genomics and Proteomics</i>. <b>1</b>(1) pp. 153-157. Doi:10.1016/j.cbd.2005.11.003]</ref>. | Tetrodotoxin, one of the most toxic biologically produced compounds known to mankind, is well-known for being produced by tetraodontidae, a family of fish known to include pufferfish and blowfish. The toxin is highly effective, presenting an LD50 of 10.7 µg/kg via injection and 532 µg/kg via ingestion in mice<ref name="Lago">[http://www.mdpi.com/1660-3397/13/10/6384 Lago Jorge, Rodriguez Laura P., Blanco Lucia, Vieites Juan Manuel, and Cabado Ana G. 2015. “Tetrodotoxin, an Extremely Potent Marine Neurotoxin: Distribution, Toxicity, Origin, and Therapeutic Uses” <i>Marine Drugs</i>. <b>13</b>(10), 6384-6406, doi:10.3990/md13106384]</ref>, and species such as Takifubu rubripes utilize the toxin actively as a self-defense mechanism <ref name="Matsumoto">[http://www.sciencedirect.com/science/article/pii/S0041010114005984 Matsumoto Takuya, Kiriake Aya, Ishizaki Shoichiro, Watabe Shugo, and Nagashima Yuji. 2015. “Biliary excretion of tetrodotoxin in the cultured pufferfish Takifugu rubripes juvenile after intramuscular administration” <i>Toxicon</i> <b>93</b>: 98-102.]</ref>. The toxin is frequently described as “over a thousand times more toxic than cyanide” <ref name="Bane">[http://www.mdpi.com/2072-6651/6/2/693/htm Bane Vaishali, Lehane Mary, Dikshit Madhurima, O’Riordan Alan, and Furey Ambrose. 2014. “Tetrodotoxin: Chemistry, Toxicity, Source, Distribution, and Detection”. <i>Toxins</i> (Basel) <b>6</b>(2): 693-755.]</ref> as a descriptor of its toxicity. However, toxic species such as these which have previously been thought to be prime producers of tetrodotoxin have been shown to become significantly less toxic when grown in non-toxic laboratory settings, indicating that production of tetrodotoxin might not be a biological process endogenous to this group [[Image:pufferfish.jpg|thumb|400px|right|A picture of a pufferfish as reported by [http://www.express.co.uk/news/world/526942/pufferfish-deadly-fish-family-supper-paralysed-fighting-for-lives-11 Rebecca Perring.]]]<ref name="Noguchi"> | ||
[https://www.researchgate.net/publication/44612067_Toxicity_of_pufferfish_Takifugu_rubripes_cultured_in_netcages_at_sea_or_aquaria_on_land Noguchi Tamao, Arakawa Osamu, Takatani Omohiro. 2006. “Toxicity of pufferfish Takifugu rubripes cultured in netcages at sea or aquaria on land.” <i>Comparative Biochemistry and Physiology Part D: Genomics and Proteomics</i>. <b>1</b>(1) pp. 153-157. Doi:10.1016/j.cbd.2005.11.003]</ref>. Many strains of bacteria have been isolated from a number of species known to produce tetrodotoxin which themselves produce tetrodotoxin, indicating that the toxicity of these macro-organisms is liable to be largely due to a mutualistic symbiotic relationship with aquatic microbes. Finally, a number of previously non-toxic tetraodontidae have been shown to become toxic when fed meals which are high in tetrodotoxin, indicating production of tetrodotoxin is likely an exogenous process<ref name="Chau">[http://www.sciencedirect.com/science/article/pii/S0166445X11000993 Chau Rocky, Kalaitzis John A., and Neilan Brett A. 2011. “On the origins and biosynthesis of tetrodotoxin” <i>Aquatic Toxicology</i>. <b>104</b>(1-2) 61-72.]</ref>. That is, the fish themselves do not seem to possess a mechanism for the production of tetrodotoxin independently. | |||
The massive degree of co-evolution which must occur in order for the host organism to exploit these microbial toxins independently indicates an altogether unique biological co-dependence between these organisms, allowing a toxin which might ordinarily be produced in too little quantities to be noticed to become dangerously relevant to humankind. Furthermore, the toxin has become so incorporated into the survival strategies of the hosts that it | The massive degree of co-evolution which must occur in order for the host organism to exploit these microbial toxins independently indicates an altogether unique biological co-dependence between these organisms, allowing a toxin which might ordinarily be produced in too little quantities to be noticed to become dangerously relevant to humankind. Furthermore, the toxin has become so incorporated into the survival strategies of the hosts that it is used in multiple ways to enhance fitness. It is not a relationship wherein the organism simply is toxic, rather, the pufferfish actively employ the toxin. Many different species of tetraodontidae accumulate variable amounts of toxin in different areas <ref name="Itoi">[http://www.sciencedirect.com/science/article/pii/S0041010113004315 Itoi Shiro, Yoshikawa Saori, Asahina Kiyoshi, Suzuki Miwa, Ishizuka Kento, Takimoto Narumi, Mitsuoka Ryoko, Yokoyama Naoto, Detake Ayumi, Takayanagi Chie, Eguchi Miho, Tatsuno Ryohe, Kawane Mitsuo, Kokubo Shota, Takanashi Shihori, Miura Ai, Suitoh Katsuyoshi, Takatani Tomohiro, Arakawa Osamu, Sakakura Yoshitaka, and Sugita Haruo. 2014. “Larval pufferfish protected by maternal tetrodotoxin” <i>Toxicon</i> <b>78</b>: 35-40.]</ref>, and even more species of organisms further implicating highly divergent evolution to take advantage of this toxin. Additionally, at least one species of predator which does not accrete or possess adaptations with which to take advantage of tetrodotoxin has been shown to have developed a resistance to the poison. This resistance is believed to have been selected through the necessity of a predator living in the same area as a toxin accreting prey species <ref name="Brodie">[http://www.jstor.org/stable/2409442?seq=1#page_scan_tab_contents Brodie III Edmund D., and Brodie Jr. Edmund D. 1990. “Tetrodotoxin resistance in garter snakes: an evolutionary response of predators to dangerous prey” <i>Evolution</i> <b>44</b>(3): 651-659]</ref>. These cement the relatively high impact of tetrodotoxin on species which come into contact with it. | ||
==Bioaccumulation and Microorganismal Role== | ==Bioaccumulation and Microorganismal Role== | ||
Tetrodotoxin has been noted to show significantly variable concentrations in various pufferfish species between species and regions. A number of studies have indicated that bioaccumulation of tetrodotoxin is exogenous to the pufferfish<ref name="Chau"></ref>. | Tetrodotoxin has been noted to show significantly variable concentrations in various pufferfish species between species and regions. A number of studies have indicated that bioaccumulation of tetrodotoxin is exogenous to the pufferfish<ref name="Chau"></ref>. Pufferfish cannot produce tetrodotoxin in isolation. Current research points toward the toxin being accumulated through the food chain, further supported by research which indicates that they are detoxified when fed nontoxic food<ref name="Noguchi2>[http://www.ncbi.nlm.nih.gov/pmc/articles/PMC2525488/ Noguchi Tamao, & Osamu Arakawa. 2008. “Tetrodotoxin – Distribution and Accumulation in Aquatic Organisms, and Cases of Human Intoxication” <i>Marine Drugs</i>, <b>6</b>(2), 220-242, doi:10.3390/md20080011]</ref>. Further, the ovaries and liver tend to contain the highest concentrations of tetrodotoxin, followed by the skin and intestines. That the intestines are one of the sites for the highest tetrodotoxin concentration sites supports the theory that these toxins are bio-accumulated through the food chain. However, at least one study posits that tetrodotoxins are variable between organisms which may point to the need to reevaluate the hypothesis that tetrodotoxins are bioaccumulated purely through the food chain<ref name="Matsumura">[http://charlie.ambra.unibo.it/didattica/docs/bioc-inq/Toxin/Marine_toxins/Production%20of%20tetrodotoxin%20in%20puffer%20fish%20embryos.pdf Matsumara, Kendo. 1998. “Production of tetrodotoxin in puffer fish embryos”. <i>Environmental Toxicology and Pharmacology</i>. <b>6</b>(4) 217-219.]</ref>. Specifically, there are 26 known analogues of tetrodotoxin<ref name="Bane"></ref>, and the mentioned study employed antibodies to tetrodotoxin to identify the compounds present in a tetraodontidae and in the prey species. Because antibodies are highly specific, the study determined that the two compounds were different between species, indicating that the food chain may not be the sole predictor of tetrodotoxin accretion<ref name="Matsumura"></ref>. | ||
[[Image:accumulation tetrodotoxin.png|thumb|600px|left|Possible pathways of tetrodotoxin accumulation as listed in a review by [http://www.mdpi.com/2072-6651/6/2/693/htm Bane et al.]]] | [[Image:accumulation tetrodotoxin.png|thumb|600px|left|Possible pathways of tetrodotoxin accumulation as listed in a review by [http://www.mdpi.com/2072-6651/6/2/693/htm Bane et al.]]] | ||
Tetrodotoxin has since been isolated as being a product of aquatic bacterial groups, leading to a high concentration of tetrodotoxin in ocean sediments<ref name="Do">[http://www.ncbi.nlm.nih.gov/pmc/articles/PMC184361/pdf/aem00085-0342.pdf Do H. K., Kogure K., and Simidu U. 1990. “Identification of deep-sea-sediment bacteria which produce tetrodotoxin”. <i>Applied and Environmental Microbiology</i>. <b>56</b>(4) 1162-1163.]</ref>. This points to a microorganismal basis of the prevalence of tetrodotoxin adaptations in higher taxa such as the tetraodontidae. Tetrodotoxin produced by microbes is accumulated in successive links in the food chain, which accounts for how both the prevalence of aquatic organisms which contain high levels of tetrodotoxin, as well as the relatively extreme concentrations of tetrodotoxin found in tetraodontidae, at the upper end of the food chain. In addition to bacteria and tetraodontidae, tetrodotoxin has been isolated from “dinoflagellates, algae, arthropods, echinoderms, molluscs, worms, newts, and frogs”<ref name="Lago"></ref>. The massive diversity of phyla which present as accreting tetrodotoxin provides additional evidence for symbiont toxin-producing bacteria being the progenitors for tetrodotoxin production<ref name="Chau"></ref> A number of bacterial species from the families Vibro, Pseudomonas, Bacillus, Aeromonas, Actinomyces, Serratia, and Microbacterium have been isolated from pufferfish as tetrodotoxin producing strains, numbering at least twenty species known to produce tetrodotoxin in total<ref name="Yu">[http://www.ncbi.nlm.nih.gov/pmc/articles/PMC3229240/ Yu Vincent Chung-Him, Yu Peter Hoi-Fu, Ho Kin-Chung, Lee Fred Wang-Fat. 2011. “Isolation and Identification of a new tetrodotoxin producting bacterial species, Raoultella terrigena, from Hong Kong Marine Puffer Fish Takifugu niphobles” <i>Mar Drugs</i>. <b>9</b>(11): 2384-2396.]</ref>, indicating that at the very least that the pufferfish provide a hospitable environment for tetrodotoxin producing microbes. However, the importance of tetrodotoxin to the bacteria themselves has yet to be determined. Further, dominance of certain microbial species in tetraodontidae differs from species to species, providing no consistent basis for the source of tetrodotoxin production in this family of organisms<ref name="Yu"></ref>. It could be that a co-evolutionary effect took place, with different species of tetraodontidae evolving alongside different microbial species which produce tetrodotoxin. However, the analogous adaptations to presence of tetrodotoxin among individual species of tetraodontidae confounds this possibility as unlikely. | Tetrodotoxin has since been isolated as being a product of aquatic bacterial groups, leading to a high concentration of tetrodotoxin in ocean sediments<ref name="Do">[http://www.ncbi.nlm.nih.gov/pmc/articles/PMC184361/pdf/aem00085-0342.pdf Do H. K., Kogure K., and Simidu U. 1990. “Identification of deep-sea-sediment bacteria which produce tetrodotoxin”. <i>Applied and Environmental Microbiology</i>. <b>56</b>(4) 1162-1163.]</ref>. This points to a microorganismal basis of the prevalence of tetrodotoxin adaptations in higher taxa such as the tetraodontidae. Tetrodotoxin produced by microbes is accumulated in successive links in the food chain, which accounts for how both the prevalence of aquatic organisms which contain high levels of tetrodotoxin, as well as the relatively extreme concentrations of tetrodotoxin found in tetraodontidae, at the upper end of the food chain. In addition to bacteria and tetraodontidae, tetrodotoxin has been isolated from “dinoflagellates, algae, arthropods, echinoderms, molluscs, worms, newts, and frogs”<ref name="Lago"></ref>. The massive diversity of phyla which present as accreting tetrodotoxin provides additional evidence for symbiont toxin-producing bacteria being the progenitors for tetrodotoxin production<ref name="Chau"></ref> A number of bacterial species from the families [i]Vibro[/i], [i]Pseudomonas[/i], [i]Bacillus[/i], [i]Aeromonas[/i], [i]Actinomyces[/i], [i]Serratia[/i], and [i]Microbacterium[/i] have been isolated from pufferfish as tetrodotoxin producing strains, numbering at least twenty species known to produce tetrodotoxin in total<ref name="Yu">[http://www.ncbi.nlm.nih.gov/pmc/articles/PMC3229240/ Yu Vincent Chung-Him, Yu Peter Hoi-Fu, Ho Kin-Chung, Lee Fred Wang-Fat. 2011. “Isolation and Identification of a new tetrodotoxin producting bacterial species, Raoultella terrigena, from Hong Kong Marine Puffer Fish Takifugu niphobles” <i>Mar Drugs</i>. <b>9</b>(11): 2384-2396.]</ref>, indicating that at the very least that the pufferfish provide a hospitable environment for tetrodotoxin producing microbes. However, the importance of tetrodotoxin to the bacteria themselves has yet to be determined. Further, dominance of certain microbial species in tetraodontidae differs from species to species, providing no consistent basis for the source of tetrodotoxin production in this family of organisms<ref name="Yu"></ref>. It could be that a co-evolutionary effect took place, with different species of tetraodontidae evolving alongside different microbial species which produce tetrodotoxin. However, the analogous adaptations to presence of tetrodotoxin among individual species of tetraodontidae confounds this possibility as unlikely. | ||
Horizontal gene transfer has been implicated as a possible reason for the prevalence of tetrodotoxin production amongst bacterial groups which may not otherwise be closely related. Further, genetic analysis has shown that the mechanism responsible for saxitoxin, which is structurally similar to tetrodotoxin, is the result of multiple horizontal gene transfer events in the microbe Anabaena circinalis<ref name="Jal">[http://onlinelibrary.wiley.com/doi/10.1111/jam.12896/full Jal S., and Khora S.S. 2015. “An overview on the origin and production of tetrodotoxin, a potent neurotoxin” <i>Journal of Applied Microbiology</i>. <b>119</b>(4): 907-916.]</ref>. Further, studies have found that a large proportion of bacteria isolated from organs known to be sites of high tetrodotoxin concentration are toxin-producing microorganisms <ref name="Wu">[http://www.sciencedirect.com/science/article/pii/S0041010105001959 Wu Zhenlong, Yang Ying, Xie Liping, Xia Guoliang, Hu Jiangchun, Wang Shujin, and Zhang Rongqing. 2005. “Toxicity and distribution of tetrodotoxin-producing bacteria in pufferfish Fugu rubripes collected from the Bohai Sea of China” <i>Toxicon</i>. <b>46</b>(4). 471-476.]</ref>. This provides evidence for the contribution of these bacteria to the toxicity of their host fish. An argument could be made that tetrodotoxin is the product or byproduct of the exposure of toxin-producing microorganisms to specific compounds which are not present in laboratory settings<ref name="Chau"></ref>. As such, the possibility that the toxicity of tetraodontidae is fueled at least in part by resident microbes cannot be completely discarded as of yet. No prevalent studies have yet been published on the toxicity of wild-caught pufferfish which are then fed a non-toxic laboratory diet. Therefore, it remains possible that toxicity stems from inoculation of fish with tetrodotoxin producing microorganisms based on their natural food systems in the wild. From there, the microbes persist inside the fish and develop the toxicity of the fish<ref name="Lago"></ref>. | Horizontal gene transfer has been implicated as a possible reason for the prevalence of tetrodotoxin production amongst bacterial groups which may not otherwise be closely related. Further, genetic analysis has shown that the mechanism responsible for saxitoxin, which is structurally similar to tetrodotoxin, is the result of multiple horizontal gene transfer events in the microbe Anabaena circinalis<ref name="Jal">[http://onlinelibrary.wiley.com/doi/10.1111/jam.12896/full Jal S., and Khora S.S. 2015. “An overview on the origin and production of tetrodotoxin, a potent neurotoxin” <i>Journal of Applied Microbiology</i>. <b>119</b>(4): 907-916.]</ref>. Further, studies have found that a large proportion of bacteria isolated from organs known to be sites of high tetrodotoxin concentration are toxin-producing microorganisms <ref name="Wu">[http://www.sciencedirect.com/science/article/pii/S0041010105001959 Wu Zhenlong, Yang Ying, Xie Liping, Xia Guoliang, Hu Jiangchun, Wang Shujin, and Zhang Rongqing. 2005. “Toxicity and distribution of tetrodotoxin-producing bacteria in pufferfish Fugu rubripes collected from the Bohai Sea of China” <i>Toxicon</i>. <b>46</b>(4). 471-476.]</ref>. This provides evidence for the contribution of these bacteria to the toxicity of their host fish. An argument could be made that tetrodotoxin is the product or byproduct of the exposure of toxin-producing microorganisms to specific compounds which are not present in laboratory settings<ref name="Chau"></ref>. As such, the possibility that the toxicity of tetraodontidae is fueled at least in part by resident microbes cannot be completely discarded as of yet. No prevalent studies have yet been published on the toxicity of wild-caught pufferfish which are then fed a non-toxic laboratory diet. Therefore, it remains possible that toxicity stems from inoculation of fish with tetrodotoxin producing microorganisms based on their natural food systems in the wild. From there, the microbes persist inside the fish and develop the toxicity of the fish<ref name="Lago"></ref>. |
Revision as of 18:59, 13 May 2016
By [Richard Dennis]
Tetrodotoxin, one of the most toxic biologically produced compounds known to mankind, is well-known for being produced by tetraodontidae, a family of fish known to include pufferfish and blowfish. The toxin is highly effective, presenting an LD50 of 10.7 µg/kg via injection and 532 µg/kg via ingestion in mice[1], and species such as Takifubu rubripes utilize the toxin actively as a self-defense mechanism [2]. The toxin is frequently described as “over a thousand times more toxic than cyanide” [3] as a descriptor of its toxicity. However, toxic species such as these which have previously been thought to be prime producers of tetrodotoxin have been shown to become significantly less toxic when grown in non-toxic laboratory settings, indicating that production of tetrodotoxin might not be a biological process endogenous to this group
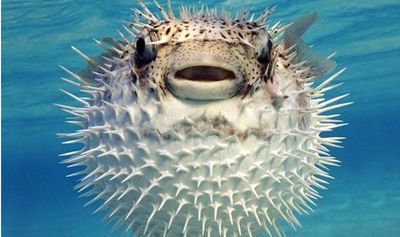
[4]. Many strains of bacteria have been isolated from a number of species known to produce tetrodotoxin which themselves produce tetrodotoxin, indicating that the toxicity of these macro-organisms is liable to be largely due to a mutualistic symbiotic relationship with aquatic microbes. Finally, a number of previously non-toxic tetraodontidae have been shown to become toxic when fed meals which are high in tetrodotoxin, indicating production of tetrodotoxin is likely an exogenous process[5]. That is, the fish themselves do not seem to possess a mechanism for the production of tetrodotoxin independently.
The massive degree of co-evolution which must occur in order for the host organism to exploit these microbial toxins independently indicates an altogether unique biological co-dependence between these organisms, allowing a toxin which might ordinarily be produced in too little quantities to be noticed to become dangerously relevant to humankind. Furthermore, the toxin has become so incorporated into the survival strategies of the hosts that it is used in multiple ways to enhance fitness. It is not a relationship wherein the organism simply is toxic, rather, the pufferfish actively employ the toxin. Many different species of tetraodontidae accumulate variable amounts of toxin in different areas [6], and even more species of organisms further implicating highly divergent evolution to take advantage of this toxin. Additionally, at least one species of predator which does not accrete or possess adaptations with which to take advantage of tetrodotoxin has been shown to have developed a resistance to the poison. This resistance is believed to have been selected through the necessity of a predator living in the same area as a toxin accreting prey species [7]. These cement the relatively high impact of tetrodotoxin on species which come into contact with it.
Bioaccumulation and Microorganismal Role
Tetrodotoxin has been noted to show significantly variable concentrations in various pufferfish species between species and regions. A number of studies have indicated that bioaccumulation of tetrodotoxin is exogenous to the pufferfish[5]. Pufferfish cannot produce tetrodotoxin in isolation. Current research points toward the toxin being accumulated through the food chain, further supported by research which indicates that they are detoxified when fed nontoxic food[8]. Further, the ovaries and liver tend to contain the highest concentrations of tetrodotoxin, followed by the skin and intestines. That the intestines are one of the sites for the highest tetrodotoxin concentration sites supports the theory that these toxins are bio-accumulated through the food chain. However, at least one study posits that tetrodotoxins are variable between organisms which may point to the need to reevaluate the hypothesis that tetrodotoxins are bioaccumulated purely through the food chain[9]. Specifically, there are 26 known analogues of tetrodotoxin[3], and the mentioned study employed antibodies to tetrodotoxin to identify the compounds present in a tetraodontidae and in the prey species. Because antibodies are highly specific, the study determined that the two compounds were different between species, indicating that the food chain may not be the sole predictor of tetrodotoxin accretion[9].
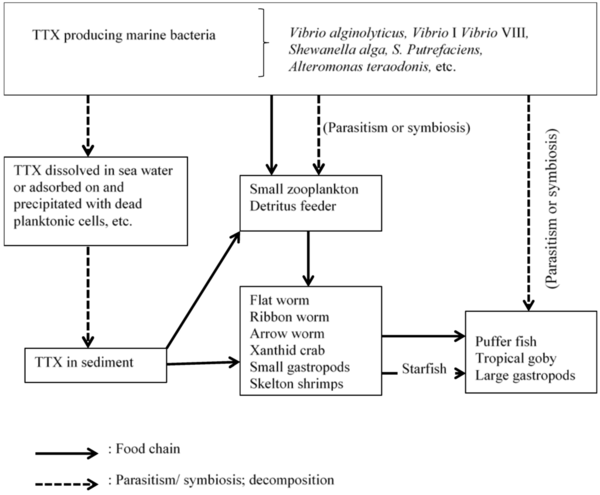
Tetrodotoxin has since been isolated as being a product of aquatic bacterial groups, leading to a high concentration of tetrodotoxin in ocean sediments[10]. This points to a microorganismal basis of the prevalence of tetrodotoxin adaptations in higher taxa such as the tetraodontidae. Tetrodotoxin produced by microbes is accumulated in successive links in the food chain, which accounts for how both the prevalence of aquatic organisms which contain high levels of tetrodotoxin, as well as the relatively extreme concentrations of tetrodotoxin found in tetraodontidae, at the upper end of the food chain. In addition to bacteria and tetraodontidae, tetrodotoxin has been isolated from “dinoflagellates, algae, arthropods, echinoderms, molluscs, worms, newts, and frogs”[1]. The massive diversity of phyla which present as accreting tetrodotoxin provides additional evidence for symbiont toxin-producing bacteria being the progenitors for tetrodotoxin production[5] A number of bacterial species from the families [i]Vibro[/i], [i]Pseudomonas[/i], [i]Bacillus[/i], [i]Aeromonas[/i], [i]Actinomyces[/i], [i]Serratia[/i], and [i]Microbacterium[/i] have been isolated from pufferfish as tetrodotoxin producing strains, numbering at least twenty species known to produce tetrodotoxin in total[11], indicating that at the very least that the pufferfish provide a hospitable environment for tetrodotoxin producing microbes. However, the importance of tetrodotoxin to the bacteria themselves has yet to be determined. Further, dominance of certain microbial species in tetraodontidae differs from species to species, providing no consistent basis for the source of tetrodotoxin production in this family of organisms[11]. It could be that a co-evolutionary effect took place, with different species of tetraodontidae evolving alongside different microbial species which produce tetrodotoxin. However, the analogous adaptations to presence of tetrodotoxin among individual species of tetraodontidae confounds this possibility as unlikely.
Horizontal gene transfer has been implicated as a possible reason for the prevalence of tetrodotoxin production amongst bacterial groups which may not otherwise be closely related. Further, genetic analysis has shown that the mechanism responsible for saxitoxin, which is structurally similar to tetrodotoxin, is the result of multiple horizontal gene transfer events in the microbe Anabaena circinalis[12]. Further, studies have found that a large proportion of bacteria isolated from organs known to be sites of high tetrodotoxin concentration are toxin-producing microorganisms [13]. This provides evidence for the contribution of these bacteria to the toxicity of their host fish. An argument could be made that tetrodotoxin is the product or byproduct of the exposure of toxin-producing microorganisms to specific compounds which are not present in laboratory settings[5]. As such, the possibility that the toxicity of tetraodontidae is fueled at least in part by resident microbes cannot be completely discarded as of yet. No prevalent studies have yet been published on the toxicity of wild-caught pufferfish which are then fed a non-toxic laboratory diet. Therefore, it remains possible that toxicity stems from inoculation of fish with tetrodotoxin producing microorganisms based on their natural food systems in the wild. From there, the microbes persist inside the fish and develop the toxicity of the fish[1].
Adaptation to Microbial Toxins
Regardless of the source of tetrodotoxin in tetraodontidae, the fact remains that it is originally formed by bacteria, and the fish are not only hosts for toxin-producing bacterial groups, but have additionally evolved in such as way so as to actively utilize tetrodotoxin when it is readily available to them. It is possible that evolution in parallel with tetrodotoxin was incidental, as a result of colonization of pufferfish by tetrodotoxin producing bacteria [14]. Specifically evolving toward a specific toxin from a prey species is not only cost-intensive, but may yield highly variable results[14], which may echo in the variable levels of tetrodotoxin between pufferfish species. This means that even if the primary source of tetrodotoxin in pufferfish are the foods they eat, the evolution of tetraodontidae to utilize the toxins defensively may have been guided by their colonization by the bacterial species which produce the toxin in the first place.
Tetraodontidae have been shown additionally to retain tetrodotoxin, compared to unrelated species which have evolved contrasting mechanisms through which they seem to detoxify tetrodotoxin, providing strong implications regarding an evolutionary pathway involving exploitation of tetrodotoxin. Further, a specific protein, known as pufferfish saxitoxin and tetrodotoxin binding protein (PSTBP) has been isolated as a product of pufferfish which assists in this retention of tetrodotoxin. Localization of this protein to the liver, skin, and ovaries of pufferfish support the high tetrodotoxin concentrations typically found in these areas[15]. The localization of the tetrodotoxin in the skin has obvious implications in terms of deterring predators, but the other areas seem less obvious in the evolutionary reason as locales for accretion of tetrodotoxin. The liver has been found to be the avenue from which the tetrodotoxin is excreted to the skin via bile from the gallbladder[15].
The most evident way this takes place is through direct excretion of tetrodotoxin through the skin of Takifubu rubripes from the liver[2], where tetrodotoxin is most concentrated in the majority of tetraodontidae. Furthermore, older members of T. rubripes were indicated to be more adept at retaining tetrodotoxin compared to younger experimental fish[2]. However, a number of other mechanisms involving creative exploitation of tetrodotoxin have been identified as well.
Further, one study found tetrodotoxin levels to actually increase in embryonic pufferfish[9]. Although no assays were done for the possible presence of tetrodotoxin producing microorganisms, tetrodotoxin levels showed a marked increase prior to hatching of pufferfish larva, implying that either these pufferfish are developing their own pathway for tetrodotoxin production, or bacteria are playing a role; embryonic fish cannot access the posited food chain explanation for tetrodotoxin bioaccumulation. Tetrodotoxin levels were found to decrease following hatching, however[9]. Whatever causes tetrodotoxin accumulation in embryos does not last beyond hatching in a laboratory setting.
Studies have indicated that embryonic and larval pufferfish actually contain some level of tetrodotoxin. This is believed to be the result of the high tetrodotoxin localization in the ovaries of tetraodontidae[6]. Furthermore, the latent tetrodotoxin levels in developing pufferfish larva was sufficient to deter juvenile fish which might ordinarily predate other larval-stage fish[6]. Beyond the obvious implications in terms of exploiting latent tetrodotoxin levels in adult pufferfish to give their offspring a competitive advantage, this additionally demonstrates that these predators must be able to sense the presence of the toxin, speaking to the high prevalence of tetrodotoxin in aquatic environments.
However, moving one step further in evolution, studies indicate that some species have evolved resistance to tetrodotoxin not just on the level of a mutualism with toxic bacteria. Predators such as Thamnophis sirtalis have evolved a degree of resistance to intoxication as a means of allowing them to predate larger numbers of animals, specifically Taricha granulosa, a prey species which secretes tetrodotoxin much like tetraodontidae[7]. Although studies show that the resistance may vary between members of T. sirtalis, this can be attributed to allopatry based on the prevalence of absence of the prey species T. granulosa. Due to the high toxicity of tetrodotoxin, it is possible that consumption of the prey species rapidly selects for organisms which are more resistant, developing a more fit predatorial species capable of resisting the effects of tetrodotoxin[7]. Compared to tetraodontidae, this is a unique case in that T. sirtalis does not even accrete tetrodotoxin. It has simply developed an independent resistance to tetrodotoxin without developing a specific adaptation.
Mechanism and Toxicity Symptoms
Tetrodotoxin acts by blocking the voltage gated sodium channels of the intoxicated organism’s nerve fibers, effectively preventing action potentials. However, the molecule is known to have no effect on the permeability of a nerve cell to potassium ions[12]. This makes it highly disruptive in the development of the charge necessary to generate an action potential, hence the paralytic symptoms. Further, the toxin binds to the sodium channel for tens of seconds, compared to the usual sodium accessing the channel which is bound reversibly for nanoseconds; the binding of a molecule of tetrodotoxin results in it closing the sodium channel for an amount of time on the order of 10 orders of magnitude longer than intended [16]. It is believed that the resistance of tetraodontidae to the effects of tetrodotoxin is due to a mutated change in the configuration of sodium channels, resulting in tetrodotoxin no longer being able to bind to the channel.
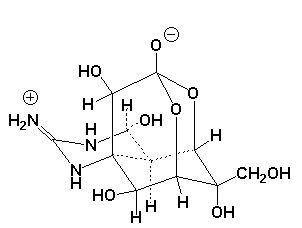
Because the toxin has an effect on such a common nerve cell expression, indeed affecting how nerve cells develop their action potentials, tetrodotoxin has been found to affect a very broad range of animals. Animals from fish to mammals are known to be affected unless specific adaptations have been made to reduce or avoid the effects of the toxin, as is the case with the pufferfish. This vulnerability is highlighted by the fact that organisms which would ordinarily be natural predators of pufferfish do not consume them, and humans themselves are frequently affected when they do eat pufferfish[6].
Tetrodotoxin intoxication has a rapid effect, with most documented cases being due to ingestion, and death typically occurring within the first four to eight hours, but sometimes within 20 minutes or as long as 24 hours following exposure. In the case of ingestion, the first stage of symptom progression begins with the numbing of the lips and tongue, before paralysis spreads to the face and extremities. Headache and dizziness, a sense of lightness or floating, copious sweating and salivation, nausea, vomiting, and diarrhea, abdominal pain, weakness and difficulty moving, and speech impairments additionally present in the first stage[17]. These symptoms then progress to increasing paralysis, spreading from the body’s extremities in toward the core, before paralysis begins to affect respiratory muscles, resulting in shortness of breath. Low blood pressure and unusual heart rhythms signal the effect of the toxin on the cardiac muscles, and dilated pupils may additionally present before possible onset of coma or seizures. Death typically follows as a result of complete paralysis of the respiratory organs resulting in respiratory failure[17]. However, the action potentials necessary for driving cardiac muscle tissue have been shown to be resistant to the effects of tetrodotoxin [18]. This explains how patients suffering from tetrodotoxin poisoning do not go under cardiac arrest even as other body parts suffer increasing paralysis. No antidote is presently available for tetrodotoxin poisoning. Anticholinesterases have been administered with little effect, largely due to the non-overlapping targets of the toxin with the drug. Although antibodies have been successful in preliminary studies at eliminating the toxicity of tetrodotoxin, further studies on the efficacy of the antibodies in vivo have not been published[3].The only effective treatment at present is to provide assistance in breathing until tetrodotoxin is completely excreted from the body[3]. In early poisoning, activated charcoal can mitigate additional absorption of the toxic compound by the body. Further, removal of small quantities of liquid from the stomach via gastric lavage may also mitigate further attempts. However, gastric lavage should only be attempted within an hour of intoxication. Unfortunately, gastric lavage is associated with a host of additional risks as well, such as not enough oxygen access, low heart beat frequency, reduced blood sodium or chloride, and nosebleed. Additional intravenous fluid administration to maintain blood electrolyte levels is important[3].
Human Relevance
Despite the drastic effects of intoxication, pufferfish is considered a delicacy in Japan, known as fugu, which occasionally results in accidental death due to consumption of toxic parts.
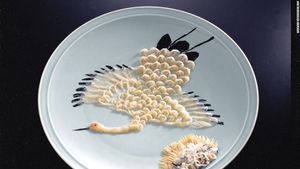
However, due to modern medicine, deaths as a result of tetrodotoxin intoxication have become increasingly rare. During an incident in Thailand in which 245 people were intoxicated, 239 made a full recovery, five died, and one suffered brain damage from lack of oxygen[3]. Given that tetrodotoxin is known to be almost completely fatal without medical intervention, this is a pretty solid survival rate.
Further, tetrodotoxin is both water-soluble and heat resistant; it actually becomes more toxic when cooked, making it a problem which cannot be solved with traditional food sterilization methods[3]. Furthermore, the continued globalization of civilizations is leading to the danger of consuming tetrodotoxin accreting organisms spreading beyond East Asian countries, with areas as far removed as Spain and the United States receiving cases of poisoning due to consumption of these fish in the late 20th and early 21st centuries[3]. However, despite there being a decent body of literature indicating that the tetraodontidae become completely non-toxic when raised in captivity without access to tetrodotoxin-containing food or tetrodotoxin developing bacteria, there are no signs of the development of a non-toxic farm raising of tetraodontidae.
Despite this, the toxin has some medicinal potential as well. Studies indicate that the paralytic effect of tetrodotoxin may be effective in relieving chronic pain [19]. Because tetrodotoxin affects voltage-gated sodium channels which are implicated heavily in pain reception, they might help mitigate the sensation of chronic pain, which affects around 20% of the adult population. Further, tetrodotoxin has been shown to be effective in the reduction of withdrawal symptoms in recovering heroin addicts. It assists with maintaining lower craving levels and anxiety upon exposure to heroin cues [20]. Research further indicates that tetrodotoxins may have therapeutic potential as a reducer of cardiac arrhythmia, and in the process indicates that selective closure of voltage gated sodium channels is sufficient to produce the desired effect on cardiac tissue[21]. Additionally, tetrodotoxin is a popular compound for neurological studies due to its unparalleled ability to block neural action potentials. It was one of the first clearly defined ion inhibitors, and is frequently the first choice in neurological studies which would benefit from isolation of certain neurological effects, or else the dampening of peripheral neurological effects[18]. Specifically, use of tetrodotoxin in studies can help eliminate the effects of miscellaneous neurological input and examine the specific spontaneous activation of neural fibers of interest. The high specificity of the toxin to voltage gated sodium channels further exemplifies the usefulness of the compound in neurological study[18].
References
- ↑ 1.0 1.1 1.2 Lago Jorge, Rodriguez Laura P., Blanco Lucia, Vieites Juan Manuel, and Cabado Ana G. 2015. “Tetrodotoxin, an Extremely Potent Marine Neurotoxin: Distribution, Toxicity, Origin, and Therapeutic Uses” Marine Drugs. 13(10), 6384-6406, doi:10.3990/md13106384
- ↑ 2.0 2.1 2.2 Matsumoto Takuya, Kiriake Aya, Ishizaki Shoichiro, Watabe Shugo, and Nagashima Yuji. 2015. “Biliary excretion of tetrodotoxin in the cultured pufferfish Takifugu rubripes juvenile after intramuscular administration” Toxicon 93: 98-102.
- ↑ 3.0 3.1 3.2 3.3 3.4 3.5 3.6 3.7 Bane Vaishali, Lehane Mary, Dikshit Madhurima, O’Riordan Alan, and Furey Ambrose. 2014. “Tetrodotoxin: Chemistry, Toxicity, Source, Distribution, and Detection”. Toxins (Basel) 6(2): 693-755.
- ↑ Noguchi Tamao, Arakawa Osamu, Takatani Omohiro. 2006. “Toxicity of pufferfish Takifugu rubripes cultured in netcages at sea or aquaria on land.” Comparative Biochemistry and Physiology Part D: Genomics and Proteomics. 1(1) pp. 153-157. Doi:10.1016/j.cbd.2005.11.003
- ↑ 5.0 5.1 5.2 5.3 Chau Rocky, Kalaitzis John A., and Neilan Brett A. 2011. “On the origins and biosynthesis of tetrodotoxin” Aquatic Toxicology. 104(1-2) 61-72.
- ↑ 6.0 6.1 6.2 6.3 Itoi Shiro, Yoshikawa Saori, Asahina Kiyoshi, Suzuki Miwa, Ishizuka Kento, Takimoto Narumi, Mitsuoka Ryoko, Yokoyama Naoto, Detake Ayumi, Takayanagi Chie, Eguchi Miho, Tatsuno Ryohe, Kawane Mitsuo, Kokubo Shota, Takanashi Shihori, Miura Ai, Suitoh Katsuyoshi, Takatani Tomohiro, Arakawa Osamu, Sakakura Yoshitaka, and Sugita Haruo. 2014. “Larval pufferfish protected by maternal tetrodotoxin” Toxicon 78: 35-40.
- ↑ 7.0 7.1 7.2 Brodie III Edmund D., and Brodie Jr. Edmund D. 1990. “Tetrodotoxin resistance in garter snakes: an evolutionary response of predators to dangerous prey” Evolution 44(3): 651-659
- ↑ Noguchi Tamao, & Osamu Arakawa. 2008. “Tetrodotoxin – Distribution and Accumulation in Aquatic Organisms, and Cases of Human Intoxication” Marine Drugs, 6(2), 220-242, doi:10.3390/md20080011
- ↑ 9.0 9.1 9.2 9.3 Matsumara, Kendo. 1998. “Production of tetrodotoxin in puffer fish embryos”. Environmental Toxicology and Pharmacology. 6(4) 217-219.
- ↑ Do H. K., Kogure K., and Simidu U. 1990. “Identification of deep-sea-sediment bacteria which produce tetrodotoxin”. Applied and Environmental Microbiology. 56(4) 1162-1163.
- ↑ 11.0 11.1 Yu Vincent Chung-Him, Yu Peter Hoi-Fu, Ho Kin-Chung, Lee Fred Wang-Fat. 2011. “Isolation and Identification of a new tetrodotoxin producting bacterial species, Raoultella terrigena, from Hong Kong Marine Puffer Fish Takifugu niphobles” Mar Drugs. 9(11): 2384-2396.
- ↑ 12.0 12.1 Jal S., and Khora S.S. 2015. “An overview on the origin and production of tetrodotoxin, a potent neurotoxin” Journal of Applied Microbiology. 119(4): 907-916.
- ↑ Wu Zhenlong, Yang Ying, Xie Liping, Xia Guoliang, Hu Jiangchun, Wang Shujin, and Zhang Rongqing. 2005. “Toxicity and distribution of tetrodotoxin-producing bacteria in pufferfish Fugu rubripes collected from the Bohai Sea of China” Toxicon. 46(4). 471-476.
- ↑ 14.0 14.1 Mebs, Dietrich. 2001. “Toxicity in animals. Trends in evolution?” Toxicon. 39(1). 87-96.
- ↑ 15.0 15.1 Yotsu-Yamashita Mari, Okoshi Natsumi, Watanabe Kouichi, Araki Nao, Yamaki Hiroe, Shoji Yuki, and Terakawa Takahiro. 2013. “Localization of pufferfish saxitoxin and tetrodotoxin binding protein (PSTBP) in the tissues of the pufferfish, Takifugu pardalis, analyzed by immunohistochemical staining” Toxicon. 72: 23-28.
- ↑ Tetrodotoxin: Mode of Action”, 2001. Chemical Ecology
- ↑ 17.0 17.1 “Tetrodotoxin: Biotoxin”. 2014. Centers for Disease Control and Prevention Accessed April 25, 2016
- ↑ 18.0 18.1 18.2 Meir, Alon. “Tetrodotoxin – A Powerful Molecular Tool in Excitable Tissues” Alomone Labs Modulator 25.
- ↑ Nieto Francisco Rafael, Cobos Enrique Jose, Tejada Miguel Angel, Sanchez-Fernandez Cristina, Gonzalez-Cano Rafael, and Cendan Cruz Miguel. 2012 “Tetrodotoxin (TTX) as a therapeutic agent for pain” Mar Drugs 10(2): 281-305
- ↑ Shi Jie, Liu Ting-Ting, Wang Xi, Epstein David H., Zhao Li-Yan, Zhang Xiao-Li, Lu Lin. 2009. “Tetrodotoxin reduces cue-induced drug craving and anxiety in abstinent heroin addicts” Pharmacology Biochemistry ad Behavior 92(4): 603-607.
- ↑ Duff Henry J., Sheldon Robert S., and Cannon Nancy J. 1988. “Tetrodotoxin: sodium channel specific anti-arrhythmic activity” Cardiovascular Research. 22(11): 800-807.
Authored for BIOL 238 Microbiology, taught by Joan Slonczewski, 2016, Kenyon College.