How E. aerogenes Affects Circadian Rhythm
Circadian Rhythm
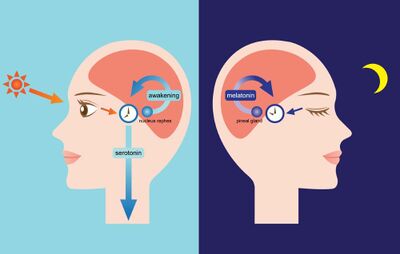
By Olivia Smith
Circadian rhythm is a natural endogenous process that regulates the sleep-wake cycle in an organism [1]. This clock cycle is driven by an internal diurnal oscillator set for a period of 24 hours [2]. Circadian rhythms regulate behavior, organs, specifically the gut, and cells in living organisms [3]. External environmental cues, including light, temperature, and redox cycles, can adjust the cycle [1]. Organisms evolved to develop regulatory clock cycles to adapt to the circadian nature of their environment [2]. To competitively survive, as well as optimize function and health, organisms have accommodated rhythmic environmental challenges [12]. Animals, bacteria, fungi, and plants all exhibit diurnal oscillations of varying degrees of complexity [1]. This page explains the bidirectional relationship between daily fluctuations of gut microbiota and its host.
The circadian rhythm is linked to the light/dark cycle [4]. The suprachiasmatic nucleus drives the light/dark cycle in the hypothalamus [2]. In mammals, the primary circadian clock is located in the suprachiasmatic nucleus in the hypothalamus [2]. The suprachiasmatic nucleus generates the clock cycle and synchronizes the periphery [2]. The cycle is synchronized to solar time by retinal afferents from intrinsically photoreceptive retinal ganglion cells [2]. The eye’s retina contains photoreceptive ganglion cells that are photoreceptive and project to the suprachiasmatic nucleus [2].
Ganglion cells contain melanopsin, a photopigment, and signals down a pathway to the suprachiasmatic nucleus [5]. The information from the retinal ganglion cells interprets the lengths of the day and night and passes it to the pineal gland in the epithalamus [9]. The pineal secretes the hormone melatonin. Melatonin secretion peaks at night and ebbs during the day [9]. Pineal melatonin feeds back to suprachiasmatic nucleus rhythmicity to modulate circadian cycles [9].
There are proteins in the suprachiasmatic nucleus that activate the transcription of genes encoding the repressors PERIOD and CRYPTOCHROME [1]. The products of these genes can form a repressive complex [1]. This PER/CRY complex can translocate into the nucleus and inhibit the suprachiasmatic nucleus proteins CLOCK and BMAL1 from transcription activity resulting in PERIOD and CRYPTOCHROME gene repression [1]. This is called the backward limb of the clock. The forward limb of the clock is when BMAL1 and CLOCK are promoted.
Sample citations:
[6]
A citation code consists of a hyperlinked reference within "ref" begin and end codes.
To repeat the citation for other statements, the reference needs to have a names: "<ref name=aa>"
The repeated citation works like this, with a forward slash.[7]
Gut microbiome on circadian rhythm
The mammalian gastrointestinal system contains a circadian clock that controls intestinal function [3]. Circadian rhythms regulate intestinal microbiota through both intrinsic clock cycles and the host organism [4]. Microbiota rhythms are regulated by diet and fasting time, which can alter metabolic activity as well as microbiota structure [4]. This can impact the host’s immune and metabolic function [4]. The gut microbiome exhibits circadian oscillation and synchronizes it with the host circadian clock [4].
Bacterial circadian rhythms are the simplest clocks, exemplified by cyanobacteria. Recent research of Synechococcus elongatus, a cyanobacteria, demonstrated that its circadian clock can be reconstituted in vitro with the Kai protein complex of its central oscillator. The first in vitro circadian rhythm was discovered by composing the proteins KaiA, KaiB, KaiC, and the energy source ATP [Nakajima et al. 2005]. Previous studies of prokaryotic circadian rhythms were said to be dependent on a DNA transcription/translation feedback loop [2]. Research on fecal microbiota exhibited a difference in the composition of the microbes depending on the time of day [5]. More than 15% of the detected microbes oscillate daily in their relative abundance [5]. These species include Clostridiales, Lactobacillales, Bacteroidales, Firmicutes, and Proteobacteria [5].
Additionally, the total biomass of the gut microbiome is higher during the active phase of the circadian rhythm for the species [5]. During the active phase, 23 % of genes for the pathways involved in energy metabolism, DNA, repair, and cell growth peak in abundance [5]. In contrast, the dark phase shows many of those genes for pathways involved in detoxification, environmental sensing, and motility [5]. These temporal changes help the bacteria anticipate changes in gastrointestinal function [5]. The relationship between daily oscillations of gut microbiota and the host is bidirectional. The host regulates the daily oscillation in the gut microbiome [5]. The daily oscillation of microbial structure requires a functional circadian clock in the host [5]. For example, if the repression of proteins CLOCK and BMAL1 is disrupted, the daily oscillations are abolished in bacterial abundance and metagenomic gene contents [5]. The forward limb of the clock can abolish the circadian rhythmicity of the gut microbiota composition if disrupted [5]. An example of the circadian rhythm disruption via jet lag can shift the gut microbiota [5]. The host circadian clock is fundamental to communicating environmental cues to the gut microbiota [5]. This will help synchronize, coordinate, and adapt between the host and bacteria [5].
Diurnally oscillating microbiota are regulated by both the host and its environment [4]. However, many circadian rhythms rely on environmental cues; they can also be dynamic and persist in the absence of these signals, such as light and temperature [1]. Host-derived melatonin and body temperature are direct regulators of gut microbiome oscillation [2]. When isolated, Enterobacter aerogenes expressed circadian behavior in swarming and motility [4]. The oscillations of E. aerogenes were enhanced by melatonin levels [4]. Melatonin is a rhythmically controlled circulating hormone [4]. The isolation of E. aerogenes proved that bacteria within the gut microbiome likely have endogenous circadian clocks that interact with the host circadian clock via signaling molecules, such as hormones [4].
Dietary components and the timing of meals plays a vital role in the circadian oscillation of the gut microbiome [4]. Diet and feeding also influence circadian rhythms of the gut microbiome [4]. High-fat diets cause a lack of daily fluctuation in the relative abundance of Bacteroidetes [4]. Restricting feeding, or fasting, during the light phase rescues the loss of the changes in the gut microbiota [4]. The circadian rhythms of host behavior are entrainable by daily cycles of restricted feeding [4]. During the active phase, the host will be eating, but during the dark phase, the host will be fasting and resting [1].
The gut microbiome also influences the peripheral host circadian rhythm [4]. Peripheral clocks exist in the host’s liver, gut, heart, and retina [4]. Metabolism, a metabolic function in both the host and the microbiome, is intertwined with circadian rhythm [4]. Evidence for oscillation of the gut microbiome and a bidirectional interaction between the gut microbiome rhythm and the host circadian clock has now been well-established [4].
Enterobacter aerogenes
Include some current research, with at least one figure showing data.
Enterobacter aerogenes on Circadian Rhythm
Include some current research, with at least one figure showing data.
Section 4
Conclusion
References
- ↑ 1.0 1.1 2017. Brain basics: Understanding sleep.
- ↑ 2.0 2.1 2.2 2.3 2.4 2.5 2.6 Ratiner, K., Elinav, E. “Basic Biology of Rhythms and the Microbiome.” 2017. Circadian Rhythms in Bacteria and Microbiomes 317-328.
- ↑ FitzGerald, G.A. “Timing the microbes: the circadian rhythm of the gut microbiome.” 2017. J Biol Rhythm 32:505-515.
- ↑ Forsyth, C.B., Green, S.J., Engen, P.A., Keshavarzian, A. “Chapter Nine - Circadian Rhythm and the Gut Microbiome.” 2016. International Review of Neurobiology.
- ↑ Wright, J.M., Patel, A.G., Cassone, V.M. "Human Gut Bacteria Are Sensitive to Melatonin and Express Endogenous Circadian Rhythmicity. 2016. PloS one.
- ↑ Bartlett et al.: Oncolytic viruses as therapeutic cancer vaccines. Molecular Cancer 2013 12:103.
- ↑ Cite error: Invalid
<ref>
tag; no text was provided for refs namedaa
Authored for BIOL 238 Microbiology, taught by Joan Slonczewski, 2022, Kenyon College