Bacterial nucleation in pseudomonas syringae
Introduction
Pseudomonas syringae
Species
As a Gram-negative, rod-shaped, obligate aerobic bacterium, Pseudomonas syringae is one of 78 species that has been described in the Pseudomonas genus (1). Characterized as an epiphyte, it grows supported non-parasitically by plants where it derives its nutrients and water from floating dust, rain, etc (9). This species can be found on tomatoes and beans to rice and tobacco, and is responsible for more surface frost damage to plants than any other mineral or organism (4). Its affiliation as a plant pathogen which causes disease in a large variety of plants makes this particular bacterium important in the field of food and biomass production, and is an important focus for the Department of Energy (2). P. syringae is a very stress-tolerant organism, and is the focus of many studies of stress-tolerant gene expression. Kurz et al., (2010) used biochemical approaches to address water stress tolerance in P. syringae (3). They showed that different osmolytes differentially contribute to water stress tolerance and interact at the level of transcription.
Different species of these flagellated, motile bacteria infect leaves and tissues of a wide range of hosts, but can be specific to a particular location of an individual. For example, Pseudomonas syringae pv. aesculi is able to infect vascular tissue to cause cankers in European horse chestnut in northwest Europe, but does not infect leaves, buds, or flowers on the same individual (Picture 2) (5). Some have also identified genes which are only expressed when the bacterium is on plants, which represent a ‘hidden genome’ not very well studied due to the absence in culture (2). Although there is variation with each pathovar, this bacterium grows optimally in cool, wet conditions from 15-25°C. It is proposed by Hirano et al. (1987) that momentum from falling raindrops is responsible for initiation of rapid growth and the forming of massive colonies on host surfaces (6). This has been seen as a method of how these bacterial cells are able to spread infection. This can also however, prevent infection by washing off bacterial cells from yet-infected surfaces.

Pseudomonas syringae shown using SEM. Source: Gordon Vrdoljak, Electron Microscopy Laboratory, U.C. Berkeley [1]
Importance
This particular species of Pseudomonas has been the subject of a large array of studies over the past 35 years with its involvement in crop freezing. The majority of frost-sensitive plants usually suffer from damage between -2 and -5°C (10, 11). When water gets this cold, water turns into ice in both inter- and intracellular ways, causing frost damage. P. syringae express a particular type of surface protein, ice-nucleation protein (INP), which increases temperatures to which water is able to freeze. In the absence of sites capable of ice nucleation, the cold water can supercool and freezing will not occur until the temperature is low enough for the most active ice nucleus available is able to catalyze crystallization of supercooled water (freezing)(12). Thus, supercooling instead of ice-nucleation could be a mechanism of frost protection.
P. syringae is a species of bacteria very important to present-day society, particularly with the ever-increasing population of planet earth. Chronic hunger affects 820 million people worldwide, killing 25,000 people every day, one child every eight seconds (13). Large quantities of crops are lost every year are lost due to frost such as in the state of Florida, where 40% of the world’s orange juice supply is grown (14). A large area of interest with Pseudomonas syringae focuses on genetically engineering these bacteria with ice-nucleation-minus proteins. Without these proteins it is thought the temperatures tolerable by plants may be decreased.
Ice Nucleation Active (INA) Proteins
Description
All proteins interact with water, but there are two classes in particular which have a function relating to ice: antifreeze proteins (AFPs) and ice-nucleation proteins (INPs). AFPs have particular structures known to inhibit formation of ice crystals, while INPs do just the opposite. INPs can be seen affiliated with the production of artificial snow (6). Pure water can be supercooled to -40°C in the absence of a heteronucleus (7). INPs are able to promote ice formation by raising the nucleation temperature, and in vitro this temperature can range from -14 to -2°C depending on the number of proteins that cluster together.
Structure
Graether and Jia (2001) attempted to present a model of INP from Pseudomonas syringae based on comparison with two newly determined insect AFP structures. They analyzed the INP sequence of ~60 16-residue repeats similar to a different model organism, and proposed a 16-residue loop for P. syringae (Picture 3). Their result suggested that insect AFPs and bacterial INPs may have a similar B-helical structure, even though they have opposite effects on water molecules.
Bacterial Effects
Beginning more than 25 years ago, scientists began noticing that concentrations of bacteria residing on leaf surfaces were correlated with the temperatures at which freezing occurred in plant tissues. A study by Lindow et al., (1982) concluded that higher concentrations of P. syringae on leaf surfaces were associated with warmer temperatures of freezing (Figure 1). They also showed that freezing temperatures were much lower without the presence of the bacteria (Figure 2). These results led researchers to conclude that bacteria on the surfaces of leaves play a major role in plant freezing.
Current Research
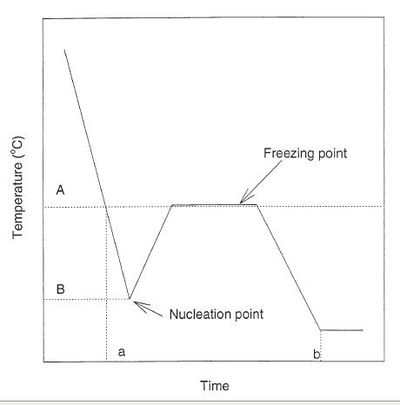
Hypothetical freezing curve of water. Degree of supercooling is defined as the temperature difference between freezing point (A) and nucleation point (B). The total freezing time is the time difference between when the temperature of water passes the freezing point (a), and when it reaches the freezer temperature (b). Source: Li et al., 1997.
Conclusion
References
[1] http://genome.jgi-psf.org/psesy/psesy.home.html [2]
[2] Steele, H., B.E. Laue, G.A. MacAskill, A.J. Hendry, and S. Green. “Analysis of the natural infection of European horse chestnut (Aesculus hippocastanum) by Pseudomonas syringae pv. Aesculi.” Plant Pathology 59: 1005-1013.
[3] Graether, S.P., and Z. Jia. 2001. Modeling Pseudomonas syringae ice-nucleation protein as a B-helical protein. Biophysical Journal 80: 1169-1173.
[4] Lindow, S.E., D.C. Arny, and C.D. Upper. 1982. Bacterial ice nucleation: a factor in frost injury to plants. Plant Physiology 70: 1084-1089.
Edited by Ryan O'Connor,student of Joan Slonczewski for BIOL 238 Microbiology, 2011, Kenyon College.