Microbial Biofuels: Current Production and Future Prospects
By Mark Michael Harden
Introduction
Fossil fuels like coal and oil have played a critical role in humanity’s recent history, providing a vast energy source which has fueled much of society’s development and industrialization. These fuels are still the primary source of energy for the world’s developed nations, and yet it is agreed that these traditional sources of energy cannot continue to power humanity’s growth into the future. The demand for oil production is at an all-time high, and will only increase as developing nations continue to grow. Furthermore, many experts predict that the rate of world oil production has already peaked, and that it will only decrease from now onwards as fewer and fewer oil reserves are discovered. This decreasing supply and rising demand will drive up the price of oil and other fossil fuels, and will eventually make them economically unsustainable [2]. The use of fossil fuels poses other problems as well, most notably that their consumption is environmentally unsustainable. Burning fossil fuels produces enormous quantities of the greenhouse gas carbon dioxide, which has a negative impact on the Earth’s environment by contributing to global warming [10, 14]. For all of these reasons, there is great incentive to pursue the development of renewable energy sources, particularly microbial biofuels.
Microbial metabolism is incredibly varied, and can both utilize and produce a wide variety of useful molecules. Many microbial systems are also well characterized and easy to manipulate genetically, and scientific advances will only make these systems easier to work with in the future [6]. Although there is no biofuel option currently available which solves all of the economic and environmental issues associated with fossil fuels, the potential for both “fine-tuning” biofuel-producing microbes, or genetically modifying species to be able to efficiently make use of otherwise useless materials and byproducts, makes microbial biofuels an appealing target for research [6].
Current Microbial Biofuels: Ethanol Produced by Fermentation
Microbial biofuel production is already in use, principally in the form of sugar fermentation by yeast to produce ethanol [4]. Although many microbes have been used in ethanol production, the yeast species Saccharomyces cerevisiae is primarily used in industry, using starch and sugars from plants as the starting material for the process [3]. The most common feedstocks (carbon source utilized by the microbes) are agricultural products which can easily be processed to create the simple sugars needed for fermentation. This is primarily corn in the United States, wheat in the European Union, and sugar cane in Brazil [4, 10].
Ethanol fermentation by S. cerevisiae is primarily done via the standard glycolysis pathway (Figure 1) [3]. In the case of corn and other starch-containing plants, the simple sugars necessary are formed via the hydrolysis of starch to yield monosaccharide subunits, whereas the sugars in sugarcane are hydrolyzed only once and then go straight into the pathway [6]. In the process, a single molecule of glucose is oxidized to two molecules of pyruvate. Anaerobic conditions are required, so that molecular oxygen is not available for use as an electron acceptor, and instead pyruvate itself must be used as the terminal electron acceptor. This involves the decarboxylation of pyruvate to form carbon dioxide and acetaldehyde, and the subsequent reduction of acetaldehyde to produce ethanol [3].
The use of fermented bio-ethanols as a fuel source offers some advantages over standard fossil fuels. Both corn ethanol and sugarcane ethanol require an input of fossil fuel energy in order to produce them because of the energy requirements of farming, processing, and transportation. However, several studies report that they yield more biofuel energy than fossil fuel energy that is invested in them, giving them a positive net energy balance (NEB), which means that they represent more efficient energy sources. The NEB for corn ethanol is relatively small, at approximately 25% extra energy output than the required fossil fuel energy investment [10]. This means that, while corn ethanol reduces the amount of necessary fossil fuel, it does not represent a total solution to the problem. Ethanol produced via sugarcane fermentation has a much higher reported NEB than corn ethanol, yielding almost eight times as much biofuel energy compared to the required input of fossil fuel energy. However, because sugarcane only grows in certain tropical climates, this also does not represent a viable solution for the worldwide problem [9].
Ethanol fermentation by yeast also helps to address the problem of greenhouse gas emissions, although it does not represent a perfect solution from an environmental perspective either. All biofuels with a positive NEB should theoretically emit less carbon dioxide, because the process of carbon fixation occurring within the growing plants should counterbalance the carbon dioxide emissions of both the invested fossil fuel energy and the combustion of ethanol [10]. However, in reality the nitrogen-rich fertilizer used to sustain the plants and the addition of extra plant matter into the soil supports communities of bacteria that produce nitrous oxide, a much more potent greenhouse gas than carbon dioxide. Considering this entire system, producing ethanol via corn fermentation emits approximately 88% of the greenhouse gas content of gasoline yielding the same amount of energy [15]. This mediocre improvement, coupled with the other environmental implications such as pesticides, make most current ethanol fermentation techniques of limited use, although they are nevertheless a positive alternative to fossil fuels.
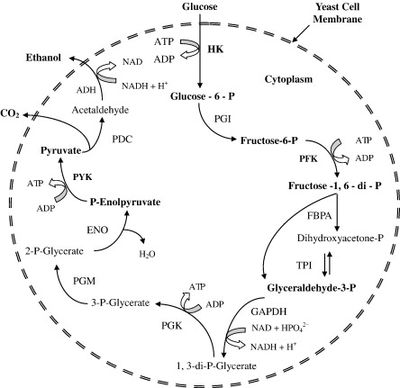
Image source: http://www.sciencedirect.com/science/article/pii/S0734975007001000 [3]
Alternative Ethanol-Producing Microorganisms: Zymomonas mobilis and Escherichia coli

Image source: http://onlinelibrary.wiley.com/doi/10.1002/bit.23021/full [1]
Alternative Feedstocks
Lignocellulose
Fatty Acids and Glycerol
Alternative Biofuels
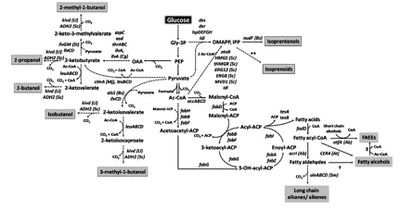
Image source: http://link.springer.com/article/10.1007/s00253-010-2446-1 [6]
Other Fermentation Products
Non-Fermentation Products
Improving Microbial Biofuel Production: Recent Developments
Using Efflux Pumps to Improve Biofuel Production
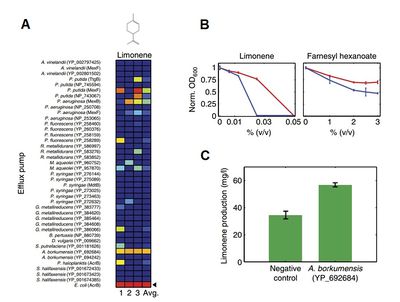
Image source: http://www.nature.com/msb/journal/v7/n1/full/msb201121.html [8]
References
Edited by student of Joan Slonczewski for BIOL 238 Microbiology, 2011, Kenyon College.