Microcystis aeruginosa and the Effects of Microcystin-LR on Ecosystems and Human Health: Difference between revisions
Line 75: | Line 75: | ||
Include some current research, with at least one figure showing data.<br> | Include some current research, with at least one figure showing data.<br> | ||
<br> | <br> | ||
Algal blooms of any kind can be dangerous to the aquatic ecosystems. When these blooms die off and decompose, the rate of oxygen consumption increases, causing hypoxic conditions. Hypoxia, or oxygen deficient conditions, can cause sharp increases in fish, plant, and invertebrate mortality rates. These blooms also cover the surface of the water, preventing the sunlight from penetrating to the bottom of the aquatic environment. The lack of incoming sunlight can lead to the death of benthic organisms and drastically altered community dynamics and structure. | |||
<br> | |||
<br> | |||
Lake Erie has had a long history of toxic cyanobacteria blooms. <i>Microcystis</i> blooms were extremely common in the 1960s due to eutrophication, but were reduced in the 1970s and 1980s when nutrient runoff was minimized. However, the water quality began to worsen again in the mid-1990s, and has continued to deteriorate since. Dissolved phosphorus has been steadily increasing since the 1990s, and scientists have found a strong positive correlation between rising phosphorus levels and microcystin concentrations in the lake. In 2011, Lake Erie experienced one of the largest algal blooms in recorded history, and they’ve worsened nearly every year since. These algal blooms have had far reaching effects, putting locals at risk and polluting drinking water. This crisis culminated in the Toledo Water Crisis, which caused 400,000 people to have no access to clean drinking water for three days because microcystin concentrations were above the safe consumption level. Scientists and activists are working with government officials and farmers to try to limit the amount of phosphorus loading into Lake Erie. | |||
<br> | |||
<br> | |||
These record-breaking blooms have had far reaching economic costs on the local community. While in the midst of a <i>Microcystis</i> bloom, it is estimated that the city of Toledo is forced to spend an addition $10,000 per day to treat and purify drinking water to make it safe for consumption. In addition, hypoxic zones and contaminated water can hurt the fishing industry, as well as recreational fishing and boating. The blooms are known to give off a rotten smell, likely driving potential tourists away from the beaches. These blooms have the potential to have devastating consequences on local economies, especially if the tourism industry is unable to bounce back. | |||
==Dangers to Ecosystems== | ==Dangers to Ecosystems== |
Revision as of 19:13, 26 April 2016
Introduction

By Lauren Michael
Double brackets: [[
Filename: PHIL_1181_lores.jpg
Thumbnail status: |thumb|
Pixel size: |300px|
Placement on page: |right|
Legend/credit: Electron micrograph of the Ebola Zaire virus. This was the first photo ever taken of the virus, on 10/13/1976. By Dr. F.A. Murphy, now at U.C. Davis, then at the CDC.
Closed double brackets: ]]
Other examples:
Bold
Italic
Subscript: H2O
Superscript: Fe3+
The term “microcystin” refers to a group of liver toxins that are produced by many species of cyanobacteria. The name microcystin comes from the genus Microcystis, one of the most widely known genera that produce this widespread toxin. Microcystis aeruginosa, a photosynthesizing freshwater cyanobacterium, is the most common producer of toxic microcystin, and is often associated with eutrophic fresh water systems, where they form widespread, toxic blooms. Microcystis aeruginosa are Gram-positive cyanobacteria that form colonies ranging from a few individuals to millions of cyanobacteria, and can group together to create blue-green layers of scum on the surface of freshwater systems. This scum blocks light from reaching the bottom of the water column, and can lead to hypoxic zones due to the overconsumption of oxygen during the decomposition of dead cyanobacterial cells.
Microcystin-LR is the most deadly microcystin, and can be dangerous to humans and animals that have long-term contact with it. Microcystin contaminated water poses a major health risk for humans and animals, and has been known to cause mass die offs in contaminated ecosystems, as well as the disruption of major food webs.[1]
Microcystin is a hepatotoxin that most often enters the body through oral ingestion, primarily targeting the liver. In addition, microcystin can promote tumor growth and concentrate in the testes of males. Microcystins are able to bioaccumulate in food webs, causing concentrations to increase at higher trophic levels. Short-term physical contact with Microcystis aeruginosa can result in skin and eye irritations and gastrointestinal disruptions. These symptoms can be heightened in those with hypersensitivity to micocystin toxins. When damaged Microcystis aeruginosa cells lyse, they release microcystins into the water, making it difficult to remove Microcystis from freshwater sources. Microcystins, and in particular, Microcystin-LR, can pose a significant threat to both humans and animals.
Microcystis aeruginosa

Microcystis aeruginosa is a species of cyanobacteria in the genus Microcystis that is commonly found in freshwater environments in temperate regions. Microcystis aeruginosa harnesses the energy from the sun through photosynthesis, producing oxygen that can be utilized by other organisms in the ecosystem. M. aeruginosa is unicellular with a cell wall that is composed of layers of lipopolysaccharides and peptidoglycan. The individual cells are small and are organized into colonies, which can often be viewed without a microscope, appearing as a blue-green scum on the surface of the water. They are planktonic, meaning that they float or drift through the water in large groups, and use gas vesicles to regulate buoyancy during times of increased light availability.[2]
Microcystis aeruginosa’s ideal conditions are 15 °C to 32 °C and a pH of 6 to 9. Generally, more toxins are produced at higher water temperatures. Blooms usually occur in the early fall or late summer and frequently occur in eutrophic freshwater systems. Microcystis aeruginosa are able to utilize gas vesicles and carbohydrates produced through photosynthesis to adjust their buoyancy and change depth in the water to access areas with ideal temperatures and higher concentrations of limiting nutrients. These cyanobacteria prefer calm water, where they are able to easily maintain their position in the water column. Periods of heavy rain are also favorable for cyanobacteria blooms as they increase nutrient runoff into lakes, streams, and reservoirs.[3] Toxin quota can vary both within species and between species, meaning that often blooms with a high toxin quota but smaller bloom biomass can be more harmful than the opposite. Much of the current research on microcystin production is focused on the cause of increased toxin quota in Microcystis aeruginosa. Research has shown that increased light availability allows toxic strains of Microcystis aeruginosa to outcompete non-toxic strains, and that the increase of shade can cause a population of Microcystis aeruginosa to gradually become less and less toxic over time.[4]
The ability of Microcystis cyanobacteria to produce toxins varies from species to species as well as within species. A combination of physical, environmental, and biological factors can lead to changes in Microcystis toxicity. Studies have shown that cyanobacteria toxicity has the ability to vary temporarily and spatially in an ecosystem. 3 Specific conditions can favor the growth of toxic cyanobacteria over non-toxic, allowing the toxic species to outcompete and increase the overall concentration of toxins in the water. Elevated temperatures are highly correlated with Microcystis blooms as well as higher nutrient concentrations, indicating that both climate change and incidences of eutrophication could create blooms with a greater concentration of toxic microcystin.[5] Variations in nutrient levels, temperature, and light levels can play a vital role in determining whether Microcystis aeruginosa will be toxic or non-toxic.
Research has also linked zebra mussels (Dreissena polymorpha) to Microcystis aeruginosa blooms. Zebra mussels are an invasive mollusk that originated in Eastern Europe and was brought to the United States in the water transported on ships. These mussels have spread throughout the Great Lakes, and have caused disruptions in the aquatic ecosystems, as well as the economy of the Great Lakes region.[6] Studies have shown that zebra mussels selectively reject toxic species of Microcystis. This could act as a selective pressure that favors toxicity in Microcystis, increasing the number of toxic cyanobacteria in the population over time. In addition, zebra mussels could eliminate competitors, allowing Microcystis aeruginosa to outcompete other species of cyanobacteria present in the community.
Nitrogen-limited conditions are associated with steep declines in toxic Microcystis aeruginosa, while high nitrogen concentrations in the water show a strong positive relationship with Microcystis growth.4
The relationship between phosphorus and Microcystis growth is still debated, as recent studies have found conflicting results. Higher levels of phosphorus runoff are positively correlated with increased toxic Microcystis growth rates in combination with increased temperatures, while non-toxic cyanobacteria are less affected. However, other studies have found that treatments containing high levels of phosphorus actually have decreased Microcystis biomass.
Due to the availability of nutrients caused by sewage discharge and excess fertilizer use, nearly all freshwater sources contain toxic cyanobacteria, though the population size is dependent on environmental conditions.5
Microcystin-LR

Microcystins are cyclic heptapeptides that show a large diversity in structure. They are hepatotoxins, or liver damaging toxins, and exert their hepatotoxicity through the inhibition of two distinct protein phosphatases, 1 and 2A. When protein phosphatase 1 and 2A are inhibited, it can lead to build up of phosphorylated proteins. This build up subsequently damages the liver and causes programmed cell death of the hepatocytes, or liver cells, eventually leading to liver failure.[7] After exposure, studies have found that 70% of the toxin is rapidly transported to the liver, with the rest usually accumulating in the kidneys and intestines. Though they mainly target the liver, they can also impact the heart, nervous system, kidneys, and gastrointestinal tracts.[8] Microcystins are not able to easily cross membranes, preventing them from entering tissues. The majority of microcystin research has been performed on mice and rats; the similarities between rodent behavior, genetics, and biology are close enough to humans that human symptoms can often be replicated in rodents, and vice versa.[9]
Microcystin toxins are unable to be broken down by eukaryotes due to their strong peptide bonds, which are resistant to hydrolytic enzymes. Once microcystins enter the body, they are transported to the bloodstream and eventually sent to the liver, due to their inability to be digested properly. They are concentrated in the liver where they impede protein phosphatases 1 and 2A, quickly causing disruption in both intracellular structure and cell control.[10] The liver plays an important role in the detoxification of microcystin-LR, and the products of this process can be found in the feces and urine of those that are exposed.9
All microcystins are composed of a seven-membered peptide ring, with two protein amino acids that are distinct for each type of microcystin toxin, and five non-protein amino acids that are universal. The name of each type of microcystin comes from the two protein amino acids that are unique to their structure. The most common and toxic microcystin is microcystin-LR, named for the protein amino acids Leucine and Arginine.[11] The biodegradation time for microcystin-LR ranges from 4 days to 15 days, according to reported data, with most studies reporting a half-life of less than a week.9
Microcystins are stable when dissolved in water, making them resistant to boiling. This resistance can make it difficult for people in developing countries to be able to safely consume water from lakes, rivers, and reservoirs.
Control of Microcystis blooms is challenging but possible. Lysed Microcystis cells can release toxins into the water. Therefore, the most effective means of treatment is removal of whole, undamaged cells. Great care is needed to control the blooms safely, as traditional water treatment is unable to remove microcystins from the water. Nanofiltration and reverse osmosis removal are effective for some microcystins, but not all. In addition, techniques such as ozonation and chlorination are effective in removing microcystins from water systems, as well as the use of potassium permanganate. Many scientists have suggested riverbank or slow sand filtration, which have both proven effective at removing cyanobacterial cells from water sources without causing the cells to lyse and release toxins. Biological filtration is useful for removing most water toxins, but is highly variable depending on environmental conditions, making it a less reliable method.[12]
One obstacle that scientists face is the difficulty of measuring total microcystin concentrations in the water. To do this, it is important to measure both free microcystin in the water as well as microcystins that are contained within cells. To do this, scientists must sonicate cyanobacterial cells to break them apart, causing them to release their stored microcystins. In addition, extraction procedures must be used to ensure that the total amount of microcystins in a water source is accounted for, rather than simply those that are free-floating in the water. Many of the current datasets on microcystin levels in drinking water do not indicate whether free microcystin or total microcystin levels were used. In addition, the equipment used for water sample collection in the field can interfere with correct microcystin concentration determination. Plastics, like bisphenol A, can contaminate microcystin measurements.
One common method for quantifying microcystin levels in water sources is the protein phosphatase bioassay method. The main benefit of this method is that it allows for multiple samples to be measured in a few hours, making it highly efficient. However, it can measure the presence of non-microcystin toxins that also inhibit protein phosphatases, and therefore does not show specificity for microcystins. Mouse bioassays are often used to quantify the toxic potential of a microcystin sample. This method is able to distinguish between neurotoxins and hepatotoxins, but is unable to detect microcystin toxins at low levels, making it difficult to test drinking water. It is also unable to identify specific types of microcystins or cyanobacterial toxins. Liquid chromatography-mass spectrometry is an easy and highly selective technique that can quickly detect and analyze different types of microcystins in a sample. Though this technique is one of the most common used to measure microcystin levels, there are numerous other methods that can be selected. This choice depends on the level of specificity and accuracy that is desired, as well as the efficiency of the method.9
Dangers to Human Health
Include some current research, with at least one figure showing data.
Algal blooms of any kind can be dangerous to the aquatic ecosystems. When these blooms die off and decompose, the rate of oxygen consumption increases, causing hypoxic conditions. Hypoxia, or oxygen deficient conditions, can cause sharp increases in fish, plant, and invertebrate mortality rates. These blooms also cover the surface of the water, preventing the sunlight from penetrating to the bottom of the aquatic environment. The lack of incoming sunlight can lead to the death of benthic organisms and drastically altered community dynamics and structure.
Lake Erie has had a long history of toxic cyanobacteria blooms. Microcystis blooms were extremely common in the 1960s due to eutrophication, but were reduced in the 1970s and 1980s when nutrient runoff was minimized. However, the water quality began to worsen again in the mid-1990s, and has continued to deteriorate since. Dissolved phosphorus has been steadily increasing since the 1990s, and scientists have found a strong positive correlation between rising phosphorus levels and microcystin concentrations in the lake. In 2011, Lake Erie experienced one of the largest algal blooms in recorded history, and they’ve worsened nearly every year since. These algal blooms have had far reaching effects, putting locals at risk and polluting drinking water. This crisis culminated in the Toledo Water Crisis, which caused 400,000 people to have no access to clean drinking water for three days because microcystin concentrations were above the safe consumption level. Scientists and activists are working with government officials and farmers to try to limit the amount of phosphorus loading into Lake Erie.
These record-breaking blooms have had far reaching economic costs on the local community. While in the midst of a Microcystis bloom, it is estimated that the city of Toledo is forced to spend an addition $10,000 per day to treat and purify drinking water to make it safe for consumption. In addition, hypoxic zones and contaminated water can hurt the fishing industry, as well as recreational fishing and boating. The blooms are known to give off a rotten smell, likely driving potential tourists away from the beaches. These blooms have the potential to have devastating consequences on local economies, especially if the tourism industry is unable to bounce back.
Dangers to Ecosystems
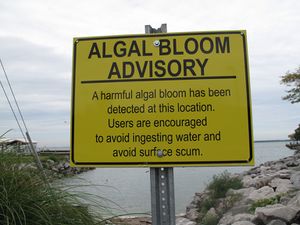
Conclusion
References
- ↑ Black, Ken, Mete Yilmaz, and Edward J. Phlips. "Growth and Toxin Production by Microcystis Aeruginosa PCC 7806 (Kutzing) Lemmerman at Elevated Salt Concentrations." Journal of Environmental Protection JEP. 02.06 (2011): 669-74. Web. 21 Apr. 2016..
- ↑ Mlouka, A., K. Comte, A.-M. Castets, C. Bouchier, and N. Tandeau De Marsac. "The Gas Vesicle Gene Cluster from Microcystis Aeruginosa and DNA Rearrangements That Lead to Loss of Cell Buoyancy." Journal of Bacteriology 186.8 (2004): 2355-365. Web. 23 Apr. 2016.
- ↑ "Cyanobacteria Toxins: Microcystin-LR in Drinking-water." World Health Organization (2003): 1-14. Who.int. World Health Organization, 2003. Web. 23 Apr. 2016.
- ↑ Horst, Geoffrey P., Orlando Sarnelle, Jeffrey D. White, Stephen K. Hamilton, Rajreni B. Kaul, and Julianne D. Bressie. "Nitrogen Availability Increases the Toxin Quota of a Harmful Cyanobacterium, Microcystis Aeruginosa." Water Research 54 (2014): 188-98. Web. 23 Apr. 2016.
- ↑ Davis, Timothy W., Dianna L. Berry, Gregory L. Boyer, and Christopher J. Gobler. "The Effects of Temperature and Nutrients on the Growth and Dynamics of Toxic and Non-toxic Strains of Microcystis during Cyanobacteria Blooms." Harmful Algae 8.5 (2009): 715-25. Web. 23 Apr. 2016.
- ↑ "Zebra Mussel (Dreissena Polymorpha)." Dnr.state.mn.us. Minnesota Department of Natural Resources, 2016. Web. 23 Apr. 2016.
- ↑ Rinehart, Kenneth L., Michio Namikoshi, and Byoung W. Choi. "Structure and Biosynthesis of Toxins from Blue-green Algae (cyanobacteria)." Journal of Applied Phycology 6.2 (1994): 159-76. Web. 23 Apr. 2016.
- ↑ Lone, Yaqoob, Raj Kumar Koiri, and Mangla Bhide. "An Overview of the Toxic Effect of Potential Human Carcinogen Microcystin-LR on Testis." Toxicology Reports 2 (2015): 289-96. Web. 23 Apr. 2016.
- ↑ "Microcystin-LR." The PubChem Project. National Center for Biotechnology Information, n.d. Web. 24 Apr. 2016.
- ↑ Falconer, Ian R., and Andrew R. Humpage. "Health Risk Assessment of Cyanobacterial (Blue-green Algal) Toxins in Drinking Water." International Journal of Environmental Research and Public Health 2.1 (2005): 43-50. Web. 23 Apr. 2016.
- ↑ "Microcystins." Cyanosite. Purdue University, n.d. Web. 23 Apr. 2016.
- ↑ "Treatment and Control Measures and Technical Achievability." World Health Organization (n.d.): n. pag. Who.int. World Health Organization. Web.
Authored for BIOL 238 Microbiology, taught by Joan Slonczewski, 2016, Kenyon College.