Microcystis aeruginosa and the Effects of Microcystin-LR on Ecosystems and Human Health
Introduction

By Lauren Michael
Double brackets: [[
Filename: PHIL_1181_lores.jpg
Thumbnail status: |thumb|
Pixel size: |300px|
Placement on page: |right|
Legend/credit: Electron micrograph of the Ebola Zaire virus. This was the first photo ever taken of the virus, on 10/13/1976. By Dr. F.A. Murphy, now at U.C. Davis, then at the CDC.
Closed double brackets: ]]
Other examples:
Bold
Italic
Subscript: H2O
Superscript: Fe3+
The term “microcystin” refers to a group of liver toxins that are produced by many species of cyanobacteria. The name microcystin comes from the genus Microcystis, one of the most widely known genera that produce this widespread toxin. Microcystis aeruginosa, a photosynthesizing freshwater cyanobacterium, is the most common producer of toxic microcystin, and is often associated with eutrophic fresh water systems, where they form widespread, toxic blooms. Microcystis aeruginosa are Gram-positive cyanobacteria that form colonies ranging from a few individuals to millions of cyanobacteria, and can group together to create blue-green layers of scum on the surface of freshwater systems. This scum blocks light from reaching the bottom of the water column, and can lead to hypoxic zones due to the overconsumption of oxygen during the decomposition of dead cyanobacterial cells.
Microcystin-LR is the most deadly microcystin, and can be dangerous to humans and animals that have long-term contact with it. Microcystin contaminated water poses a major health risk for humans and animals, and has been known to cause mass die offs in contaminated ecosystems, as well as the disruption of major food webs.[1]
Microcystin is a hepatotoxin that most often enters the body through oral ingestion, primarily targeting the liver. In addition, microcystin can promote tumor growth and concentrate in the testes of males. Microcystins are able to bioaccumulate in food webs, causing concentrations to increase at higher trophic levels. Short-term physical contact with Microcystis aeruginosa can result in skin and eye irritations and gastrointestinal disruptions. These symptoms can be heightened in those with hypersensitivity to micocystin toxins. When damaged Microcystis aeruginosa cells lyse, they release microcystins into the water, making it difficult to remove Microcystis from freshwater sources. Microcystins, and in particular, Microcystin-LR, can pose a significant threat to both humans and animals.
Microcystis aeruginosa

Microcystis aeruginosa is a species of cyanobacteria in the genus Microcystis that is commonly found in freshwater environments in temperate regions. Microcystis aeruginosa harnesses the energy from the sun through photosynthesis, producing oxygen that can be utilized by other organisms in the ecosystem. M. aeruginosa is unicellular with a cell wall that is composed of layers of lipopolysaccharides and peptidoglycan. The individual cells are small and are organized into colonies, which can often be viewed without a microscope, appearing as a blue-green scum on the surface of the water. They are planktonic, meaning that they float or drift through the water in large groups, and use gas vesicles to regulate buoyancy during times of increased light availability.[2]
Microcystis aeruginosa’s ideal conditions are 15 °C to 32 °C and a pH of 6 to 9. Generally, more toxins are produced at higher water temperatures. Blooms usually occur in the early fall or late summer and frequently occur in eutrophic freshwater systems. Microcystis aeruginosa are able to utilize gas vesicles and carbohydrates produced through photosynthesis to adjust their buoyancy and change depth in the water to access areas with ideal temperatures and higher concentrations of limiting nutrients. These cyanobacteria prefer calm water, where they are able to easily maintain their position in the water column. Periods of heavy rain are also favorable for cyanobacteria blooms as they increase nutrient runoff into lakes, streams, and reservoirs. Toxin quota can vary both within species and between species, meaning that often blooms with a high toxin quota but smaller bloom biomass can be more harmful than the opposite. Much of the current research on microcystin production is focused on the cause of increased toxin quota in Microcystis aeruginosa. Research has shown that increased light availability allows toxic strains of Microcystis aeruginosa to outcompete non-toxic strains, and that the increase of shade can cause a population of Microcystis aeruginosa to gradually become less and less toxic over time.
The ability of Microcystis cyanobacteria to produce toxins varies from species to species as well as within species. A combination of physical, environmental, and biological factors can lead to changes in Microcystis toxicity. Studies have shown that cyanobacteria toxicity has the ability to vary temporarily and spatially in an ecosystem.3 Specific conditions can favor the growth of toxic cyanobacteria over non-toxic, allowing the toxic species to outcompete and increase the overall concentration of toxins in the water. Elevated temperatures are highly correlated with Microcystis blooms as well as higher nutrient concentrations, indicating that both climate change and incidences of eutrophication could create blooms with a greater concentration of toxic microcystin. Variations in nutrient levels, temperature, and light levels can play a vital role in determining whether Microcystis aeruginosa will be toxic or non-toxic.
Research has also linked zebra mussels (Dreissena polymorpha) to Microcystis aeruginosa blooms. Zebra mussels are an invasive mollusk that originated in Eastern Europe and was brought to the United States in the water transported on ships. These mussels have spread throughout the Great Lakes, and have caused disruptions in the aquatic ecosystems, as well as the economy of the Great Lakes region. Studies have shown that zebra mussels selectively reject toxic species of Microcystis. This could act as a selective pressure that favors toxicity in Microcystis, increasing the number of toxic cyanobacteria in the population over time. In addition, zebra mussels could eliminate competitors, allowing Microcystis aeruginosa to outcompete other species of cyanobacteria present in the community.
Nitrogen-limited conditions are associated with steep declines in toxic Microcystis aeruginosa, while high nitrogen concentrations in the water show a strong positive relationship with Microcystis growth.4
The relationship between phosphorus and Microcystis growth is still debated, as recent studies have found conflicting results. Higher levels of phosphorus runoff are positively correlated with increased toxic Microcystis growth rates in combination with increased temperatures, while non-toxic cyanobacteria are less affected. However, other studies have found that treatments containing high levels of phosphorus actually have decreased Microcystis biomass.
Due to the availability of nutrients caused by sewage discharge and excess fertilizer use, nearly all freshwater sources contain toxic cyanobacteria, though the population size is dependent on environmental conditions.5
Microcystin-LR
Include some current research, with at least one figure showing data.

Dangers to Human Health
Include some current research, with at least one figure showing data.
Dangers to Ecosystems
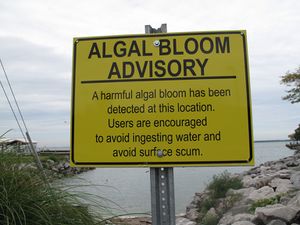
Conclusion
References
- ↑ Black, Ken, Mete Yilmaz, and Edward J. Phlips. "Growth and Toxin Production by Microcystis Aeruginosa PCC 7806 (Kutzing) Lemmerman at Elevated Salt Concentrations." Journal of Environmental Protection JEP. 02.06 (2011): 669-74. Web. 21 Apr. 2016..
- ↑ Mlouka, A., K. Comte, A.-M. Castets, C. Bouchier, and N. Tandeau De Marsac. "The Gas Vesicle Gene Cluster from Microcystis Aeruginosa and DNA Rearrangements That Lead to Loss of Cell Buoyancy." Journal of Bacteriology 186.8 (2004): 2355-365. Web. 23 Apr. 2016.
Authored for BIOL 238 Microbiology, taught by Joan Slonczewski, 2016, Kenyon College.