Nitrogen Cycle: Difference between revisions
Line 77: | Line 77: | ||
Nitrification is a two-step process of ammonium (NH4) to nitrate (NO3) by soil bacteria11. Ammonium is initially oxidized to nitrite by chemoautotrophs in the following manner NH3 + O2 --> NO2- + H+ + H2O | Nitrification is a two-step process of ammonium (NH4) to nitrate (NO3) by soil bacteria11. Ammonium is initially oxidized to nitrite by chemoautotrophs in the following manner NH3 + O2 --> NO2- + H+ + H2O | ||
The chemoautotrophs are able to use carbon dioxide as a carbon source for the oxidation of ammonium. The most common bacteria that oxidizes ammonium is Nitrosomonas.The second step of the nitrification process is the conversion of nitrite to nitrate | The chemoautotrophs are able to use carbon dioxide as a carbon source for the oxidation of ammonium. | ||
The most common bacteria that oxidizes ammonium is Nitrosomonas.The second step of the nitrification process is the conversion of nitrite to nitrate | |||
NO2- + O2 --> 2NO3- + 2H+ + 2e- | NO2- + O2 --> 2NO3- + 2H+ + 2e- | ||
The most common group of bacteria that convert nitrite to nitrate are in the genus Nitrobacter. This group of soil bacteria obtain their energy from the ammonium oxidation process. Nitrite can be toxic to certain plants, thus the incomplete conversion of nitrite to nitrate may prevent plant growth in those | The most common group of bacteria that convert nitrite to nitrate are in the genus Nitrobacter. This group of soil bacteria obtain their energy from the ammonium oxidation process. Nitrite can be toxic to certain plants, thus the incomplete conversion of nitrite to nitrate may prevent plant growth in those areas. It is important to ensure that nitrification occurs efficiently in food production systems as to prevent crop damage13. | ||
===Denitrification=== | ===Denitrification=== |
Revision as of 19:58, 13 March 2016
Introduction
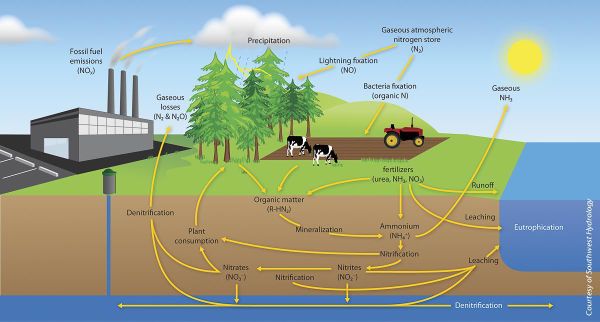
Nitrogen is one of the most abundant elements on Earth - it makes up 78% of the atmosphere, 16% of all protein diets [1], and is present at a concentration of 7.5 kilograms for every 250 kilograms of human feces [2].
The different forms of nitrogen undergo various chemical and physical transformations that are all equally critical to the global nitrogen cycle. Over the last few decades, excessive fertilizer and fossil fuel usage have lead to serious environmental problems, which have increased disease and pollution. Some of these issues include nitrate-contaminated groundwater, eutrophication, and increased production of carbon dioxide, methane, and other harmful greenhouse gases in the carbon cycle [3].
Accordingly, researchers have focused on balancing the cost to the benefits of human activities. As part of the global nitrogen cycle, soil is heavily studied not only because a significant amount of nitrogen is stored in the soil [3], but also because the increasing human population demands more nitrogen for food production.
In soil, most of the chemical transformations of nitrogen are due to microbial activities, such as nitrification and nitrogen fixation. Learning how organisms behave in soil is critical for humans to understand the complex nitrogen cycle.
1. Nitrogen cycle processes
1.1 Natural Soil Nitrogen Cycle
Dinitrogen is an essential element for the nitrogen cycle. The symbiotic microbes fix the dinitrogen into volatile ammonia, NH3, by the process of nitrogen fixation. The ammonia is further protonated to ammonium, NH4+, which is uptaken by plants to manufacture amino acids for growth. When plants decompose, the organic molecules present in the plant residues become a source of nitrogen and other nutrients to soil microbes. The microbes conduct mineralization (ammonification) to utilize the organic molecules as electron donors, acquiring energy and producing ammonium. In contrast, the transformation of organic nitrogen to ammonium is reversible through immobilization (assimilation) when the carbon to nitrogen ratio (C/N ratio) is high [4]. Ammonium can also undergo nitrification to produce NO3- in an aerobic environments. The NO3- can be utilized by plants or by other organisms in anaerobic environments as an electron acceptor, can leach out by dissolving in water, or be reduced to dinitrogen gas via denitrification processes in anaerobic conditions. During the denitrification and nitrification processes nitrous oxide, N2O, is produced and escapes into atmosphere.
1.2 Anthropogenic Influences on the Soil Nitrogen Cycle
One major influence of human input is reduced biological nitrogen fixation. With excessive ammonium input, plants no longer need the symbiotic microbes to provide ammonium. As a result, the degree of symbiosis will be diminished. Furthermore, the excessive ammonium provides nutrients for nitrifiers to produce nitrate, causing an increase in the amount of leached nitrate. Another negative outcome of anthropogenic inputs is the increased emission of nitrous oxide into the atmosphere due to increased nitrification and denitrification from excessive ammonium inputs [3].
2. Nitrogen fixation
Prokaryotes(bacteria) are responsible for the nitrogen fixation inside the soil. They mostly establish associative relationships with leguminous plants and other plant species [5]. Yet, there are free-living bacteria like Azotobacter [6]. All of the prokaryotes that are capable of nitrogen fixation have nitrogenase, which can fix nitrogen into ammonia. Many prokaryotes share the same enzyme complex through horizontal gene transfer via plasmids or evolutionary events [6]. ==2.1 Nitrogen Fixation Mechanism The overall reaction catalyzed by nitrogenase is N2 + 8H+ + 8e- + 16 ATP = 2NH3 + H2 + 16ADP + 16 Pi [7]. In the process, nitrogen is used to replace a pair of hydrogen molecules on the nitrogenase complex, which has been heavily reduced along with protonation. The rest of the H+ will bond to the nitrogen and reform the structure of the molecule. Then, H+ continues to be added to the complex and binds with an NH group to form NH3. Each complex is able to produce two NH3 molecules in each cycle of this process [7]. Once NH3 leaves the nitrogenase complex, it will be rapidly protonated to NH4+.
Atmospheric nitrogen (N2) is abundant, but unavailable for plant and microbail growth due to the high energy required to break the triple bond. In nitrogen fixation, atmospheric nitrogen is reduced to biologically useful ammonia (NH3) by the activity of prokaryotes utilizing nitrogenase. Species such as Azotobacter, Bradyrhizobium and Rhizobium have the ability to fix nitrogen. Azotobacter are free living organisms, while Bradyrhizobium and rhizobium live mutually with plants.
The reaction catalyzed by nitrogenase is N2 + 8H+ + 8e- ---> 2NH3 + H2. Ammonia produced in this way is rapidly protonated to NH4+. Nitrogenases are irreversibly inhibited by exposure to O2, and thus organisms must take steps to prevent this. Some organisms, such as Azotobacter protect their nitrogenases with a thick capsule that slows oxygen diffusion. Others, such as the cyanobacteria Nostoc form special cells with thick cell walls to exclude oxygen, allowing the cell to provide fixed nitrogen to its neighbors. Alternative nitrogenases do exist that are not deactivated by oxygen, but they are found in a relatively small number of organisms.
Key microorganisms
Nitrogen fixed by prokaryotes constitutes the vast majority of all biologically active nitrogen on the planet. Diazotrophs is the category of all organisms that are capable of fixing nitrogen (Sylvia et al., 2005). Nitrogen-fixers may be aerobic or anaerobic, and may be free-living or in a symbiotic relationship with a plant. One type of symbiotic bacteria, now collectively known as rhizobia, are found in root nodules on legumes. They use the constant source of carbon from the legumes to be able to fix nitrogen. There are other symbiotic relationships including: Ferns and cyanobacteria, Fungi and cyanobacteria, and Frankia (an actinomycete) which can be in a relationship with a variety of plants (Sylvia et al., 2005). Free living diazotrophs, like Azotobacter, are able to live in an array of environments because of their independence from plants.
Nitrogen Ammonification
Net nitrogen mineralization is commonly defined as the sum of concurrent ammonium production and consumption processes[9]. However, in recent years, it has been shown that mineralization also is driven by depolymerization of nitrogen containing polymers available to use both by plants and microorganisms[11], and thus it is more correct to call the process ammonification or gross nitrogen mineralization.
The new paradigm regarding nitrogen cycling rethinks mineralization as the center point in the nitrogen cycle[11], and instead regards depolymerization of nitrogen containing polymers as what regulates the nitrogen cycle. Ammonification or gross nitrogen mineralization is the conversion of organic-nitrogen polymers to ammonium, mediated by heterotrophic microbes. The microbial degradation of amino acids, sugars, and nucleic acids is seen as a need for energy and carbon, with ammonium released as a byproduct of catabolism[9]. Since the polymers are not immediately bioavailable due to their large size, they are first cleaved by extracellular enzymes[11].
The extracellular enzymes are created by microorganisms, and have the capacity to depolymerize proteins, aminopolysaccharides found in cell walls, nucleic acids, and to hydrolyze urea[9]. Proteins are broken down by a wide variety of proteinases (large proteins), proteases, and peptidases that cleave dipeptides or split individual amino acids1. Some examples of isolated proteolytic enzymes are subtilism, clostripain, and thermolysin[9].
Several common extracellular polymers degrade cell wall polymers, producing an end product of individual amino sugar monomers[9]. In fungi which contain primarily chitin cell walls, chitinase degrades the cell walls into dimers, and then chitobiase breaks down each dimer into N-acetylglucosamine[9]. In bacteria containing peptidoglycan cell walls, several enzymes, the most predominant of which is lysozyme, breaks the beta linkages holding the polymer together[9].
Nucleic acids are degraded by RNases and DNases, which hydrolyze ester ester bonds between phosphate and pentose sugars that make up the backbone of DNA and RNA, resulting in an end product of individual nucleotides[9].
Ureases hydrolyze urea into carbon dioxide and ammonium, which plays a key role in immediate fertilization plant availability[9]. The cofactor of the urease enzyme is nickel[9].
The research on extracellular enzymes and soil constituents has revealed some insight into the complexity of the soil matrix. An enzyme and substrate may bind to clay surfaces, protecting them from degradation and resulting in a slow degradation of the substrate over time[9]. In addition, an enzyme can remain active and act on an available substrate if its catalytic site is unaffected and structure unaltered by adsorption to the clay surface[9].
Intracellular enzymes play a critical role in nitrogen transformations[9]. In most cases, the final product of ammonium occurs as a result of intracellular enzymes within microbial cells. There are two types of nitrogen found in amino acids: amine and amide. In the amide, the asparagine and glutamate are cleaved by aspariginase and glutaminase. In the amine, amino acid dehydrogenases and oxidases release the amino nitrogen in a process called deamination.
Amino sugars are metabolized in two steps - kinase phosphorylation followed by deamination[9]. The degradation of nucleotides and subsequent ammonium release involves multiple steps - nucleotides are hydrolyzed to produce nucleosides and phosphate (PO43-), the nucleosides are then further hydrolyzed to purines and pyrimidine bases and pentose sugar molecule parts, and finally the ammonium is released during the catabolism of the purines and pyrimidines with a urea intermediate[9].
Key microorganisms
The groups of microorganisms responsible for ammonification are very broad and cover a wide range of species.
Organoheterotrophs are an example of a group of microorganisms that mineralize nitrogen, often going after carbon in addition to the nitrogenous compounds present in the plant residue.
Nitrogen Immobilization
Immobilization is the conversion of ammonium and nitrate to organic nitrogen, primarily as a result of the assimilation of ammonium by the microbial biomass.The process requires energy for the conversion of nitrate to ammonium and subsequent incorporation of ammonium into amino acids.
Key microorganisms
Heterotrophic microbes and other organisms assimilate ammonium to build up biomass.
C/N Ratios
A carbon to nitrogen ration is the ratio of the mass of organic carbon to the mass of total nitrogen in soil or organic matter (Sylvia et al. 2005) C/N ratios dictate whether the nitrogen gets immobilized or mineralized. If the C/N ratio is less than 20 than the nitrogen will get mineralized. At C/N ratios >30 the process of immobilization will occur (Scow 2008).
If organisms have an excess of nitrogen than they will excrete NH4+ back into the soil, returning nitrogen back into the system (Scow 2008). If they are nitrogen depleted they will take nitrogen out from the soil leading to nitrogen deficiencies in the soil.
Nitrification
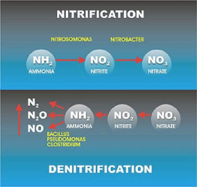
Nitrification is a two-step process of ammonium (NH4) to nitrate (NO3) by soil bacteria11. Ammonium is initially oxidized to nitrite by chemoautotrophs in the following manner NH3 + O2 --> NO2- + H+ + H2O
The chemoautotrophs are able to use carbon dioxide as a carbon source for the oxidation of ammonium.
The most common bacteria that oxidizes ammonium is Nitrosomonas.The second step of the nitrification process is the conversion of nitrite to nitrate
NO2- + O2 --> 2NO3- + 2H+ + 2e-
The most common group of bacteria that convert nitrite to nitrate are in the genus Nitrobacter. This group of soil bacteria obtain their energy from the ammonium oxidation process. Nitrite can be toxic to certain plants, thus the incomplete conversion of nitrite to nitrate may prevent plant growth in those areas. It is important to ensure that nitrification occurs efficiently in food production systems as to prevent crop damage13.
Denitrification
Denitrification is important in the continuation of the nitrogen cycle, as it is the step where gaseous dinitrogen is released back into the atmosphere. The natural cycle of denitrification comprises a series of enzymes such as bacterial NORs enzymes reduce nitrate to dinitrogen12. As a result, reduction of nitrates to gaseous dinitrogen follows a series of respiratory biochemical reactions by microorganisms12. Bacteria involved in denitrification are facultative anaerobic organoheterotrophs such as Bacillus11. The overall process of denitrification is as follows:
NO3- ------ > NO2- ------> NO ---------> N2O-------> N2
Nitrate -----> Nitrite ----> Nitric Oxide ----> Nitrous Oxide ------> Dinitrogen gas
Environmental Impacts
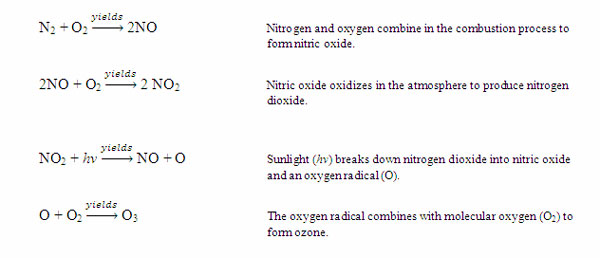
The nitrogen cycle causes many environmental issues such as global warming and pollution itself. The global increase in temperature is mainly due to the release of greenhouse gases. Greenhouse gases causes the blanket of air surrounding the earth to thicken, thus warming up the earth. The byproducts of the nitrogen cycle produce greenhouse effects, specifically in agricultural fields. Farmers input high amounts of ammonium nitrate to fertilize their soil to grow their crops. Consequently this increases the production of pollutants: nitrous oxide (N2O) and nitric oxide (NO), through soil microbial processes. CO2, another greenhouse gas, would also be produced from aerobic respiring microbes.
Excess nitrogen fixation has caused a lot of pollution into the biosphere. For example, nitrogen dioxide is degraded through photolysis to form oxygen radicals, which will react with molecular oxygen to form ozone in the troposphere, as shown in Figure 6. While ozone is beneficial in the stratosphere, where it can block UV radiation, ozone in the troposphere is a pollutant and can cause oxidative stress to humans and other organisms.
Mineralized nitrogen compounds in soil can be nitrified into nitrates, which can be leached into groundwater and cause contamination. Nitrate is soluble in water due to its negative charge; this polarization prevents adsorption onto the negatively charged soil surface and allows the soluble nitrate to leach into groundwater. Nitrate pollution is a major concern because of its many adverse effects such as methemoglobinemia, cancer, and eutrophication. Nitrification not only produces nitrates but also produces nitric acid, which will acidify bodies of water and harm sensitive marine life. Excessive nitrogen has also negatively affected terrestrial life by increasing the prevalence of pathogens, such as bacteria, viruses, and fungi. These pathogens were once limited by the lack of nitrogen but now have become more abundant.
Strategies to Lessen Environmental Impact
Some strategies to reduce mobile nitrogen in the biosphere are bioremediation, reduction of emissions, and sequestration. Bioremediation can reduce the amount of nitrate from the environment by converting it into atmospheric nitrogen and nitric oxide, or alternatively we can use dissimilatory nitrate reduction to ammonium (DNRA) to reduce nitrates back to ammonia/ammonium. However, this has its own concerns because nitric oxide production must be limited to lessen effects of global warming. Reduction of emissions reduces the amount of nitrogen entering the biosphere, thus lessening the amount of mobile nitrogen available. Sequestration of nitrogen prevents nitrogen from becoming mobile and can be increased by selecting for crops with higher nitrogen uptake efficiency that are also able to form symbiotic relationships with nitrogen sequestering soil microorganisms. There has been renewed interest in studying these relationships in the attempt to increase nitrogen sequestration in soil.
Current Research
The Center of Limnology is currently studying the rise in organic nitrogen availability to microbes. Their findings may lead to an analysis of the consequences of anthropogenic nitrogen enrichment watersheds. To learn more visit their website at http://cires.colorado.edu/limnology/research/
The nitrogen fertilizers cause environmental problems associated with leeching into our water systems and production of greenhouse gas. To decrease the usage of the fertilizers,the alternative ways to increase nitrogen available to plants are investigated. Nodulation process forming of symbiosis between nitrogen-fixation bacteria and plant is one of the interested research nowadays. Recent research has shown the evidences that the genes, called symbiosis-receptor-kinase-gene (SMYRK), are involved in a genetic program that links arbuscular mycorrhiza (AM) and one form of bacterial nodule symbiosis. Moreover, the analysis of SYMRK in several species of plant provided the evidence that the functions of SYMRK constituted an important step in the evolution of intracellular nodule symbiosis. Most plants have a short version of SMYRK, which is required for AM symbiosis. A longer variant of SMYRK was found only in plants involved in the symbiotic relationships with nitrogen-fixing bacteria. Importantly, the longer version was found in both legumes which form symbioses with rhizobia and in actinorhiza which form symbiotic relationships with Frankia bacteria, about which there is little genetic information. The results therefore suggest a common evolutionary origin of intracellular root symbioses with nitrogen-fixing bacteria (Markmann et al., 2008).
Another interesting research find regarding the nitrogen cycle is that the application of road salts can severely disrupt the nitrogen cycle(Green et al., 2008). There is evidence to suggest that sodium reduces the cation exchange capacity of ammonium reducing the ammonium concentration from the soil (Green et al., 2008).
References
1. Sylvia, D.M., Fuhrmann, J.J., Hartel, P.G.,& Zuberer, D.A. (2005). Principles and application of soil microbiology. 2nd edition.Pearson. New Jersey.
3. "Green House Gases." The Environmental Literacy Council. 2002. Last Updated May 2007. http://www.enviroliteracy.org/article.php/428.html
4. Scow, K (2008). Nitrogen Cycle. Soil Microbiology Class lecture.
5. Dalhgren, R (2007). Topic 3 Lecture on Nutrient Cycling.
6. Green S., and Cresser M., (2008) Nitrogen Cycle Disruption through the Application of De-icing Salts on Upland Highways. Water, Air, and Soil Pollution volumes 188 numbers 1-4:pages 139-153
7. Richter, A.; Daims, H.; Reigstad, L.; Wanek, W.; Wagner, M.; Schleper,. "Archaeal Nitrification in Hot Springs" American Geophysical Union, Fall Meeting 2006, abstract #B13E-06 http://adsabs.harvard.edu/abs/2006AGUFM.B13E..06R
8. ScienceDaily (Jun. 13, 2003) "Changing Global Nitrogen Cycle Impacting Human Health, Says Colorado University-led Study" http://www.sciencedaily.com/releases/2003/06/030613074458.htm Site Visited March 21st.
9. http://toxics.usgs.gov/topics/rem_act/nitrate_remediation.html
10. http://www.umich.edu/~lehnert/denitrification.html
Edited by student of Kate Scow