Phyllosphere
Introduction
The phyllosphere is a term used in microbiology to refer to leaf surfaces or total above-ground surfaces of a plant as a habitat for microorganisms. All plants are host to numerous and diverse communities of microorganisms including bacteria, fungi, and yeasts. Some are beneficial to the plant, others function as plant pathogens and may damage the host plant or even kill it. However, the majority of bacterial colonists on any given plant have no noticeable effect on plant growth or function.
Conservative estimates indicate that the roughly 1 billion square kilometers of worldwide leaf surfaces host more than 10^26 bacteria, which are the most abundant colonizers of this habitat (1, 2). The overall microbiota in this ecosystem is thus sufficiently large to have an impact on the global carbon and nitrogen cycles. Additionally, the phyllosphere inhabitants influence their hosts at the level of the individual plants.
Microbes that live in the Phyllosphere are called [Epiphytes]
Physical environment
The leaf surface has long been considered a hostile environment for bacterial colonists. The leaf surface is exposed to rapidly fluctuating temperature and relative humidity, as well as repeated alternation between presence and absence of free moisture due to rain and dew. The leaf also provides limited nutrient resources to bacterial colonists. Such rapid and extreme fluctuations in the physical conditions of above ground plant surfaces yields a hostile microbe environment.
Several factors may influence the microhabitat experienced by bacteria on leaves. First, the leaf itself is surrounded by a very thin laminar layer in which moisture emitted through stomata may be sequestered, thereby alleviating the water stress to which epiphytes are exposed.
Second, some cells in a leaf bacterial population, particularly in plant-pathogenic populations, may not reside in exposed sites on the leaf surface but instead may at least locally invade the interior of the leaf, avoiding the stresses on the exterior of the leaf by residing in substomatal chambers or other interior locations (78, 118). Thus, while some phytopathogens may have the option of avoiding stresses, most other epiphytes apparently must tolerate them in some way.
The surfaces of most plants are very tortuous at the small scales at which interactions with bacteria will occur. Epidermal cells produce bulges and troughs that will determine the shape and size of low areas on the surface, which in turn will influence the shape and spread of water droplets on the plant (77). The first contact between immigrating bacteria and a leaf normally occurs at the plant cuticle. This waxy layer, which has different three-dimensional crystalline structures on different plant species and can change as leaves age, presumably in part due to microbial modifications, limits passive diffusion of nutrients and water vapor from the plant interior onto the surface and defines the hydrophobicity of the leaf. Thick waxy cuticles have thus been thought to interfere with bacterial colonization of plants by limiting diffusion of nutrients and inhibiting the wetting of the leaf surface.
Because the Phyllosphere is a hostile environment for the residing microorganisms physical parameters contribute to stressful conditions, such as UV radiation, temperature shifts, and the presence of reactive oxygen species. Adaptation to stressful conditions was reflected by the detection of various proteins, assigned to diverse bacterial genera and detected in all analyzed samples. Among these proteins were superoxide dismutase, catalase, DNA protection proteins, chaperones, and proteins involved in the formation of the osmoprotectant trehalose.
Biological interactions
Certain bacteria can increase the wettability of leaves by producing compounds with surfactant properties (16, 43, 86). Bunster et al. (16) found that this ability occurred in 50% of the Pseudomonas strains tested. Because of the hydrophobic nature of the cuticle, it is likely that increased wettability of these habitats allows solubilization and diffusion of substrates, making them more readily available to epiphytic bacteria. Alternatively, biosurfactants may facilitate the movement of bacteria on the phylloplane, as was suggested for tolaasin, a toxin produced by Pseudomonas tolaasi (43). The water film created by the surfactant could spread the bacteria across the leaf surface to areas where nutrients are more abundant. Thus, the production of biosurfactants may be one trait by which bacteria can alter their habitat to exploit it more efficiently.
Subsection 2
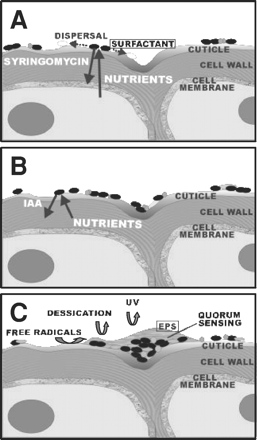
communities in the phyllosphere are thought to be limited by carbon availability, and it may be expected that access to carbon compounds on leaves is a major determinant of epiphytic colonization (2). There is evidence that small amounts of nutrients, such as simple sugars including glucose, fructose, and sucrose, leach from the interior of the plant
Microbial processes
There is viable evidence that bacteria form large and heterogeneous aggregates on plant surfaces. Microscopic examinations of colonized leaves revealed that on plant surfaces, many epiphytes occur in large mixed-bacterial-species aggregates that also harbor fungi . While large numbers of solitary bacterial cells occur on plants, a few large masses of apparently mixed bacterial species can be found.
Subsection 1
Such aggregates can constitute between 30 and 80% of the total bacterial population on certain plant species (72; J.-M. Monier and S. E. Lindow, unpublished data). These assemblages, with an extent and structure similar to those of biofilms that develop in aquatic habitats, are probably found only on long-lived leaves in moist climates such as the tropics or wet temperate regions such as the Pacific Northwest.
Subsection 2
The conglomerates on most other plants, while still sizable, are best known as aggregates. The formation of aggregates by bacteria on plants has major implications for the ability of these microbes to colonize and survive the harsh environment of the phyllosphere; it may provide them with a means to modify their immediate environment in the habitat . The production of extracellular polysaccharide (EPS), which is considered to form a major part of the bacterial aggregate matrix, may benefit epiphytes in the phyllosphere
Subsection 2a
Given that water availability is likely one of the most highly fluctuating factors on leaf surfaces, the heavy EPS slime within aggregates may shield the bacteria from desiccation stress by buffering the matric and osmotic potentials of their surroundings. Additionally, EPS has a role in protecting plant-associated bacteria from reactive oxygen species, which are often encountered on plants. It has been demonstrated that aggregated bacteria resist oxidative stress better than planktonic bacteria.
Key Microorganisms
What kind of microbes do we typically find in this environment? Or associated with important processes in this environment? Describe key groups of microbes that we find in this environment, and any special adaptations they may have evolved to survive in this environment. Add sections/subsections as needed. Look at other microbe listings in MicrobeWiki. Are some of the groups of microbes from your environment already described? Create links to those pages. Specific microbial populations will be included in the next section.
Subsection 1
Subsection 1a
Subsection 1b
Subsection 2
Examples of organisms within the group
List examples of specific microbes that represent key groups or are associated with important processes found in this environment. Link to other MicrobeWiki pages where possible.
Current Research
Research into the characteristics of microbial life in the phyllosphere is of great commercial importance to the agricultural industry for two reasons. First, understanding the survival of plant disease-causing bacteria and fungi is vital for developing new ways to control their spread. Second, there has been a recent rise in the number of food poisoning cases associated with fruit and vegetables contaminated with bacteria such as Salmonella and E. coli O157:H7. This is particularly true of fresh fruits and salads which are not cooked prior to consumption. Preventing these outbreaks by developing better decontamination strategies is important to protect public health.
References
Edited by student of Angela Kent at the University of Illinois at Urbana-Champaign.