Pseudomonas and Bioremediation: Difference between revisions
No edit summary |
|||
Line 26: | Line 26: | ||
===Aromatic hydrocarbons=== | ===Aromatic hydrocarbons=== | ||
Petroleum hydrocarbons are a major toxic contaminant in the marine environment. Two to ten million tons of crude petroleum oil are spilled annually into marine environments. The biodegradability of petroleum components generally decreases in the following order: n-alkanes, branched-chain alkanes, branched alkenes, low molecular weight ¬n-alkyl aromatics, monoaromatics, cyclic alkanes, polycyclic aromatic hydrocarbons. <i>Pseudomonas</i>strains are capable of degrading petroleum hydrocarbons and aromatic hydrocarbons such as benzene, toluene, ethylbenzene, and xylene. | Petroleum hydrocarbons are a major toxic contaminant in the marine environment. Two to ten million tons of crude petroleum oil are spilled annually into marine environments. The biodegradability of petroleum components generally decreases in the following order: n-alkanes, branched-chain alkanes, branched alkenes, low molecular weight ¬n-alkyl aromatics, monoaromatics, cyclic alkanes, polycyclic aromatic hydrocarbons. <i>Pseudomonas</i>strains are capable of degrading petroleum hydrocarbons and aromatic hydrocarbons such as benzene, toluene, ethylbenzene, and xylene. | ||
[[Image:smallputidaimage.png|thumb|300px|right|<b>Figure 2. The metabolic pathways for aromatic compounds in <i>Pseudomonas putida</i></b>. Enzyme [a], naphthalene dioxygenase, catabolizes the formation of naphthalene cis-1,2dihydrodiol from naphthalene, which then undergoes [e], meta-cleavage. Enzymes involved in other pathways are: [g], benzyl alcohol dehydrogenase; [h], benzaldehyde dehydrogenase. Square brackets indicate wide-substrate specificity enzymes involved in various pathways.]] | |||
===Polycyclic aromatic hydrocarbons=== | ===Polycyclic aromatic hydrocarbons=== | ||
Poycyclic aromatic hydrocarbons (PAH), organic chemicals consisting of three or more benzene rings, have the lowest biodegradability. They are especially problematic because of their carcinogenic potential and their tendency to persist in an environment due to their low water solubility. The Environmental Protection Agency (EPA) has listed 16 PAHs as priority pollutants. A 2014 study found that the elevated PAH concentrations after the Deepwater Horizon oil spill, which spilled 636 million liters of crude oil into the Gulf of Mexico, negatively impacted the cardiac function of resident fish species. Humans can ingest PAHs through cooked foods and the EPA has listed seven PAHs as probable human carcinogens. | Poycyclic aromatic hydrocarbons (PAH), organic chemicals consisting of three or more benzene rings, have the lowest biodegradability. They are especially problematic because of their carcinogenic potential and their tendency to persist in an environment due to their low water solubility. The Environmental Protection Agency (EPA) has listed 16 PAHs as priority pollutants. A 2014 study found that the elevated PAH concentrations after the Deepwater Horizon oil spill, which spilled 636 million liters of crude oil into the Gulf of Mexico, negatively impacted the cardiac function of resident fish species. Humans can ingest PAHs through cooked foods and the EPA has listed seven PAHs as probable human carcinogens. | ||
<i>Pseudomonas</i> strains also have the metabolic potential to degrade PAHs such as naphthalene, acenaphthene, anthracene, fluoranthene, and phenanthrene, giving them high bioremediation potential. The metabolism of naphthalene, a higher molecular weight PAH, by <i>Pseudomonas putida</i> can be used to exemplify PAH catabolism. High-molecular weight PAHs, such as naphthalene, are more recalcitrant and possess even lower biodegradability than other petroleum components. <i>Pseudomonas putida</i> uses dioxygenase enzymes (naphthalene dioxygenase, Figure 2 [a]) to catabolize the formation of cis-1,2dihydrodiol from naphthalene. Not shown in Figure 2, an iron-sulfur flavoprotein reductase accepts electrons from NADPH and transfers them to a ferredoxin. Ferredoxin reduces the terminal iron-sulfur protein which catabolizes the oxidation of naphthalene to form naphthalene cis-1,2dihydrodiol (seen in Figure 2, as the first compound after [a]). This diol is further oxidized by dehydrogenase enzymes to catechol, which enters the tricarboxylic acid cycle after meta-cleavage (Figure 2 [e]). <i>Pseudomonas putida</i> is capable of utilizing naphthalene as its sole source of carbon and energy through this metabolic pathway. Alternatively, it can hydroxylate the side-chain, leading to the eventual detoxification of naphthalene. | |||
<i>Pseudomonas</i> strains also have the metabolic potential to degrade PAHs such as naphthalene, acenaphthene, anthracene, fluoranthene, and phenanthrene, giving them high bioremediation potential. The metabolism of naphthalene, a higher molecular weight PAH, by <i>Pseudomonas putida</i> can be used to exemplify PAH catabolism. High-molecular weight PAHs, such as naphthalene, are more recalcitrant and possess even lower biodegradability than other petroleum components. <i>Pseudomonas putida</i> uses dioxygenase enzymes (naphthalene dioxygenase, Figure 2 [a]) to catabolize the formation of cis-1,2dihydrodiol from naphthalene. Not shown in Figure 2, an iron-sulfur flavoprotein reductase accepts electrons from NADPH and transfers them to a ferredoxin. Ferredoxin reduces the terminal iron-sulfur protein which catabolizes the oxidation of naphthalene to form naphthalene cis-1,2dihydrodiol (seen in Figure 2, as the first compound after [a]). This diol is further oxidized by dehydrogenase enzymes to catechol, which enters the tricarboxylic acid cycle after meta-cleavage (Figure 2 [e]). <i>Pseudomonas putida</i> is capable of utilizing naphthalene as its sole source of carbon and energy through this metabolic pathway. Alternatively, it can hydroxylate the side-chain, leading to the eventual detoxification of naphthalene. | |||
==Research Applications== | ==Research Applications== |
Revision as of 17:35, 24 March 2015
According to the Center for Climate and Energy Solutions, petroleum supplies 32.5% of global energy use (Center for Climate and Energy Solutions). The global transport of petroleum has resulted in oil spills in marine ecosystems, such as the highly publicized Deepwater Horizon oil spill off the Gulf of Mexico, the Prestige oil spill in Spain, and the Exxon Valdez oil spill in Alaska. Oil spills are costly and ecologically damaging as polycyclic aromatic hydrocarbons have been linked to cardiac arrhythmia in marine organisms. There is a great need for efficient and ecologically friendly remediation strategies.
Bioremediation is a strategy that uses naturally occurring organisms to break down pollutants, such as petroleum hydrocarbons, into less toxic substances. Species in the bacterial genus Pseudomonas present high potential for hydrocarbon degradation due to their metabolic diversity, their abundance in microbial communities, and their resistance to chemical remediation agents present at contamination sites.
Introduction
Classification
Domain: Bacteria
Phylum: Proteobacteria
Class: Gammaproteobacteria
Order: Pseudomonadales
Family: Pseudomonadaceae
Genus: Pseudomonas
General Characteristics
Pseudomonas is a genus of Gram-negative, rod-shaped bacteria. They are aerobic and non-sporulating with one or more polar flagella for motility. There are currently 218 species assigned to Pseudomonas and the genus has considerable heterogeneity (LPSN). Pseudomonas contains a number of scientifically and medically studied bacteria, such as the opportunistic pathogen P. aeruginosa, and there is increasing availability of Pseudomonas strain genome sequences. They are widespread in nature, inhabiting soil and marine habitats as well as plant and animal tissue. Pseudomonas is well known for its metabolic versatility, being able to utilize an unusually wide range of organic compounds. Certain members of the genus are able to metabolize pollutants, including P. fluorescens, P. putida, P. cepacia, P. vesicularis, and P. paucimobilis . As a result, they are often isolated and studied for their bioremediation capabilities.
Response to Oil Contamination
Pseudomonashas shown robust response to oil contamination both in vitro and in situ. A 2013 study found that Pseudomonaswas able to respond to new oil contamination by increasing its abundance and changing its community structure. Microbial community differentiation was observed in the Pseudomonas genus in response to oil contamination.
In a metagenomic analysis of the microbial population in petroleum pipelines, Pseudomonas stutzeri was detected as the most abundant hydrocarbon degrading organism. Pseudomonas stutzeri’s relative abundance was seen as a result of its ability to utilize aromatic hydrocarbons such as xylene, toluene, phenol, as well as polycyclic aromatic hydrocarbons, such as naphthalene. Researchers were able to amplify the genes for the enzymes used in the degradation of hydrocarbons from Pseudomonas stutzeri. These enzymes include: benzoate dioxygenase, toluene 1,2-dioxygenase, and catechol 1,2-dioxygenase, which play a role in aromatic hydrocarbon degradation, and methanol dehydrogenase, which is involved in aliphatic hydrocarbon degradation.
Metabolism
Aromatic hydrocarbons
Petroleum hydrocarbons are a major toxic contaminant in the marine environment. Two to ten million tons of crude petroleum oil are spilled annually into marine environments. The biodegradability of petroleum components generally decreases in the following order: n-alkanes, branched-chain alkanes, branched alkenes, low molecular weight ¬n-alkyl aromatics, monoaromatics, cyclic alkanes, polycyclic aromatic hydrocarbons. Pseudomonasstrains are capable of degrading petroleum hydrocarbons and aromatic hydrocarbons such as benzene, toluene, ethylbenzene, and xylene.
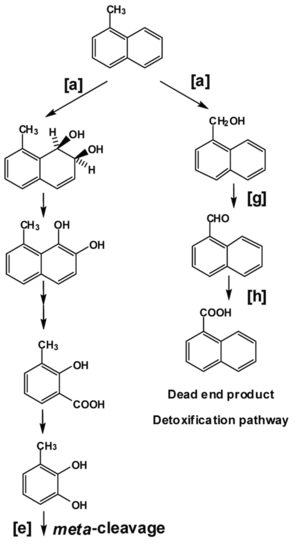
Polycyclic aromatic hydrocarbons
Poycyclic aromatic hydrocarbons (PAH), organic chemicals consisting of three or more benzene rings, have the lowest biodegradability. They are especially problematic because of their carcinogenic potential and their tendency to persist in an environment due to their low water solubility. The Environmental Protection Agency (EPA) has listed 16 PAHs as priority pollutants. A 2014 study found that the elevated PAH concentrations after the Deepwater Horizon oil spill, which spilled 636 million liters of crude oil into the Gulf of Mexico, negatively impacted the cardiac function of resident fish species. Humans can ingest PAHs through cooked foods and the EPA has listed seven PAHs as probable human carcinogens.
Pseudomonas strains also have the metabolic potential to degrade PAHs such as naphthalene, acenaphthene, anthracene, fluoranthene, and phenanthrene, giving them high bioremediation potential. The metabolism of naphthalene, a higher molecular weight PAH, by Pseudomonas putida can be used to exemplify PAH catabolism. High-molecular weight PAHs, such as naphthalene, are more recalcitrant and possess even lower biodegradability than other petroleum components. Pseudomonas putida uses dioxygenase enzymes (naphthalene dioxygenase, Figure 2 [a]) to catabolize the formation of cis-1,2dihydrodiol from naphthalene. Not shown in Figure 2, an iron-sulfur flavoprotein reductase accepts electrons from NADPH and transfers them to a ferredoxin. Ferredoxin reduces the terminal iron-sulfur protein which catabolizes the oxidation of naphthalene to form naphthalene cis-1,2dihydrodiol (seen in Figure 2, as the first compound after [a]). This diol is further oxidized by dehydrogenase enzymes to catechol, which enters the tricarboxylic acid cycle after meta-cleavage (Figure 2 [e]). Pseudomonas putida is capable of utilizing naphthalene as its sole source of carbon and energy through this metabolic pathway. Alternatively, it can hydroxylate the side-chain, leading to the eventual detoxification of naphthalene.
Research Applications
Genetic Bioaugmentation
The metabolic versatility of Pseudomonas is reflected in its large genome size, typically above 6 Mb. Horizontal gene transfer via plasmids, phages, and transposons has been reported in Pseudomonas, which aids in the distribution of the genus’s diverse metabolic pathways. The metabolic capacities of Pseudomonas can be further enhanced and exploited through genetic bioaugmentation in order to develop more efficient bioremediation strategies.
Pseudomonas putidaexhibits the metabolic capability to degrade naphthalene, a recalcitrant high-molecular weight PAH. The genes for naphthalene dioxygenase from Pseudomonas putida are encoded on the NAH7 plasmid and the nucleotide sequence for the genes is publicly available (Simon et al. 1992). Furthermore, researchers have cloned and shown the expression of naphthalene dioxygenase activity in E. coli (Ensley et al 1987). The successful transfer of naphthalene degradation ability shows the potential of genetic bioaugmentation strategies in Pseudomonas.
Compatibility with Chemical Remediation
Dispersants are commonly used spill-treating agents which promote the formation of smaller oil droplets. This increases the surface area to volume ratio of the oil droplet and allows a greater number of hydrocarbon degrading bacteria to come into direct contact with the oil.
Dispersants influence the biodegradation rate by bacteria. In a study to evaluate the inhibitory effects on the growth of Pseudomonas sp., the experimental results revealed that dispersants were not significantly toxic to Pseudomonas sp. As a gram-negative bacteria, Pseudomonas sp. was less sensitive to spill treating agents than gram-positive bacteria. The negligible sensitivity of Pseudomonas sp. to the STAs tested in the study make it an ideal organism to accompany chemical remediation. These results were obtained from in vitro studies, so the effect of STAs should be further assessed on the evolution of a bacterial community in seawater.
At right is a sample image insertion. It works for any image uploaded anywhere to MicrobeWiki. The insertion code consists of:
Double brackets: [[
Filename: Pseudomonas putida metabolic pathway.png
Thumbnail status: |thumb|
Pixel size: |300px|
Placement on page: |right|
Legend/credit: Electron micrograph of the Ebola Zaire virus. This was the first photo ever taken of the virus, on 10/13/1976. By Dr. F.A. Murphy, now at U.C. Davis, then at the CDC.
Closed double brackets: ]]
Other examples:
Bold
Italic
Subscript: H2O
Superscript: Fe3+
Overall paper length should be 3,000 words, with at least 3 figures with data.
Further Reading
[Sample link] Ebola Hemorrhagic Fever—Centers for Disease Control and Prevention, Special Pathogens Branch
References
Edited by Jessica Cheng, a student of Suzanne Kern in BIOL168L (Microbiology) in The Keck Science Department of the Claremont Colleges Spring 2015.