Quasispecies Theory and Novel HIV Therapeutics Based on Lethal Mutagenesis
A Viral Biorealm page on the family Quasispecies Theory and Novel HIV Therapeutics Based on Lethal Mutagenesis
The human immunodeficiency virus (HIV) is the causative agent of acquired immunodeficiency syndrome (AIDS), the most common cause of death secondary to infection globally. As of the writing of this wiki, HIV is estimated to have infected more than 75 million people globally and has been the proximate cause of death of 36 million people [1,2].
Since the first reported cases of AIDS and the discovery of HIV as its cause in the early 1980's, researchers and clinicians have struggled to develop and administer effective therapeutics to combat HIV/AIDS, and the production of a viable vaccine remains an unrealized goal [3]. The difficulty of developing effective HIV therapeutics and vaccines is due largely to the extraordinary mutation rate of HIV, which enables the virus to rapidly evade the selective pressures imposed by antiretroviral medications and potential vaccines by generating a large and genetically diverse population through mutagenesis [4]. A comprehensive knowledge of the genetic diversity characteristic of HIV populations in infected individuals - what have been termed viral quasispecies - is therefore essential for the discovery and delivery of effective HIV medications and vaccines.
Novel therapeutics based on fundamental components of quasispecies theory, most notably the concepts of error catastrophe and lethal mutagenesis, have been developed and constitute a promising approach toward an effective cure for AIDS and the global eradication of HIV.
Quasispecies Theory and HIV
The near-infinite population sizes, high rates of mutagenesis, and rapidity of replication of RNA viruses preclude their description by classical population genetics, in which the fitness of a specific phenotype is principally the product of its ability to reproduce successfully [5]. In RNA viruses such as HIV, extremely high rates of error-prone mutation during the course of viral replication create large swarms of genetically diverse variants. Collectively, this swarm of similar-yet-divergent variants, which stems from an original infection event by a founder RNA virus, forms a quasispecies [6]. Within a quasispecies, phenotypic fitness depends on both an individual variant's reproductive ability and its capacity for mutation. Understanding the link between quasispecies theory and its effect on the intrahost behavior of HIV may help elucidate therapeutic strategies targeting the attenuation or elimination of retroviral pathogenesis in infected individuals [7].
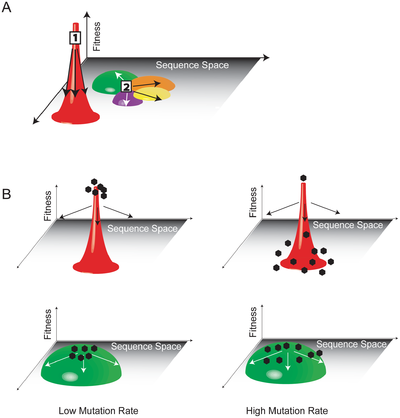
To be considered a quasispecies, a virus must meet the following criteria:
1. Individual variants within the population must have diverged through mutation from the master sequence of a founder virus.
2. Individual variants within the population must respond interactively to selective pressures within the host.
3. Each variant must contribute to the larger identity and attributes of the total population [7].
Genetic Diversity and HIV Quasispecies
The immense genetic diversity present within its quasispecies is one of the main characteristics of HIV infection within a host. The great majority of HIV infections stem from the transmission of a single virion - the master sequence around which the quasispecies is organized [8]. The generation of HIV quasispecies in infected hosts is driven principally by the low replicative fidelity of HIV reverse transcriptase and the rapid kinetics of viral replication. Reverse transcription of the HIV RNA genome results in 1-2 mutations per virion, or about 10-4 mutations at each transcribed nucleotide [7,9]. Current evidence suggests that, within a given RNA viral quasispecies, at least every possible point mutation and a large number of double mutations are produced during each cycle of replication [10]. The vast genetic diversity of the resulting HIV quasispecies has important implications for viral fitness. Cellular and tissue tropism, immune escape, and virulence are significant determinants of viral fitness in addition to replicative capacity [11]. The ability to produce an expansive array of genetic variants - to probe a large area of sequence space (a geometric model of sequence variation in which genetic divergence is represented by physical distance) - allows HIV to rapidly adapt to selective pressures, infect a wide breadth of cells, and reproduce successfully.
Mutational Coupling
In an HIV quasispecies, the phenomenon of mutational coupling allows for the persistence of genetic diversity at the expense of the replicative ability of some individual variants. Mutational coupling occurs when a variant with a relatively low replicative ability persists because of its proximity in sequence space to a variant with a relatively higher replicative ability [12]. Variants deemed unfit according to traditional population genetics may nevertheless exhibit high fitness if they are likely to undergo mutations that will produce de novo variants with greater replicative ability (their mutational couple). The consequences of mutational coupling on the course of HIV infection have been depicted graphically. In Figure #, the "fitness landscape" of a quasispecies is represented as containing peaks (high fitness, smaller area of sequence space), valleys (low fitness, larger areas of sequence space), and intermediate areas of relative flatness. Quasispecies with a high degree of mutational coupling are better able to occupy greater areas of sequence space - and achieve a higher overall degree of fitness - than those confined to discrete peaks in the fitness landscape. The robust mutagenesis and corresponding high degree of mutational coupling in HIV enables its effective occupation of sequence space and facilitates its ability to propagate within infected hosts [7].
Error Threshold and Error Catastrophe
Although HIV quasispecies are well-suited to occupy large swathes of sequence space through the rapid generation of mutant virions, a trade-off exists between the genetic diversity actually expressed within a host and the virus' ability to adapt to selective pressures. The small size (9 kilobases long), complex protein products, and the presence of three open reading frames of the HIV genome all limit the amount of viable variants that may be replicated in successive generations. There is a high likelihood that significantly deleterious mutations will arise from each cycle of HIV replication [9,13]. The 'error threshold' of a viral quasispecies describes the maximum rate of mutation at which the quasispecies can persist within a host without becoming overcome by deleterious mutations and diverging permanently from the master sequence [14]. Experimental evidence on the effect of induced mutagenesis in vesicular stomatitis virus (VSV) and HIV suggests that RNA viruses produce mutations at rates very near their error thresholds [15]. Of particular interest, a study by Crotty et al. examined the effects of the endogenous anti-HIV mutagen class APOBEC3 and exogenous mutagens on the adaptive fitness of HIV in vivo. Crotty and colleagues found that even slight increases in HIV mutational rate, induced by any of the mutagens used, severely attenuated the virus' replicative ability and resulted in the rapid accumulation of non-functional virions; HIV progression beyond the replicative error threshold resulted in error catastrophe, the collapse of viral fitness [16]. These results imply that HIV infection may be effectively treated by stimulating the rate of viral mutation, a strategy known as lethal mutagenesis [17].
Endogenous and Exogenous Mutagens Induce HIV Lethal Mutagenesis
APOBEC3
The human APOBEC3 proteins are cytokines which induce lethal mutagenesis in retroviruses. The best-studied of these cytokines is APOBEC3G, which combats HIV infection in primary CD4+ T lymphocytes [18]. APOBEC3G incorporates into budding virions in HIV infected cells and interferes with viral replication upon the subsequent infection of neighboring T cells [19]. Following the production of single-stranded, negative-sense DNA by reverse transcriptase, APOBEC3G deaminates cytidine bases in the viral DNA, producing a uracil base at each deamination site. Uracil-studded DNA is subsequently targeted for degradation by endogenous apurinic/apyrimidinic endonuclease and uracil N-glycosylase or is transcribed to a hyper-mutated positive-sense DNA strand that produces excessive stop codons within HIV genomic open reading frames. The hyper-mutated virions generated following the successful mutagenic action of APOBEC3G cross the error threshold and are rendered non-viable by deleterious mutations and error catastrophe [20-22].
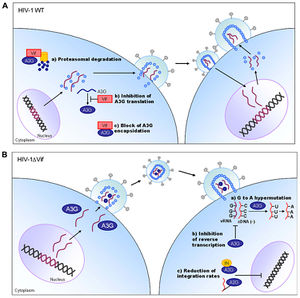
The lethal mutagenic activity of APOBEC3G illustrates a compelling approach for the control of HIV infectivity and viability. Indeed, several studies have reported that APOBEC3 cytokines is capable of decreasing HIV infectivity by 90% within one cycle of replication [23-25]. APOBEC3 lethal mutagens are not without their limitations, however. A 2009 study by Jern and colleagues implicated APOBEC3 cytokines in the induction of sub-lethal mutagenesis during HIV replication, which may ultimately accelerate the adaptation of HIV to selective pressures (i.e. HIV therapeutics) and stimulate drug resistance [26]. Additionally, the endogenous activity of APOBEC3 cytokines is limited by the activity and adaptation of the HIV Vif accessory protein, which inhibits the antiviral action of APOBEC3 by stimulating its degradation in proteasomes [22]. Although the APOBEC3 cytokines exert a strong selective pressure against HIV via lethal mutagenesis, these endogenous antiretrovirals are nevertheless insufficient to combat HIV infection by themselves. Fortunately, exogenous mutagens have been developed which are effective against HIV infection in preclinical evaluations.
5-AZC
5-AZC (5-azacytidine) is a ribonucleoside analog that has been demonstrated to limit the infectivity of HIV [26]. In infected cells, 5-AZC stimulates HIV mutagenesis during the reverse transcription of negative-sense viral DNA. First, 5-AZC-diphosphate is reduced by endogenous ribonucleotide reductase to 5-aza-2'-deoxycytidine diphosphate (5-aza-dCDP), which is then phosphorylated to yield 5-aza-2'-deoxycytidine triphosphate (5-aza-tCDP). 5-aza-tCDP is incorporated into the negative-sense viral DNA during transcription and undergoes spontaneous cytosine ring-opening and deformylation, producing a guanylurea compound [27]. This guanylurea compound then base pairs with deoxycytidine (C) deoxyribonucleosides. Following the integration of mutated viral DNA into the host genome, 5-aza-tCDP is removed from the viral positive-sense DNA by endogenous DNA machinery and replaced with guanosine (G) nucleosides. During the subsequent transcription of viral RNA, the newly-inserted G nucleosides effect G-to-C transversion mutations in the synthesized positive-sense viral RNA [28]. The extensive G-to-C transversion mutations lead to error catastrophe and the collapse of HIV's replicative ability.
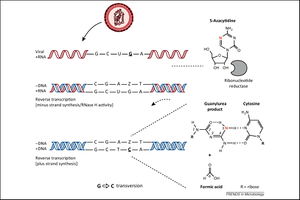
Decitabine and Gemcitabine
Two clinically-approved drugs for the treatment of cancer, decitabine (5-aza-2'-deoxycytidine) and gemcitabine (2'-deoxy-2', 2'-difluorocytidine), have been demonstrated to induce HIV lethal mutagenesis. Decitabine is a cytosine analog that induces G-to-C transversion mutations by a manner similar to that of 5-AZC. Gemcitabine is an irreversible ribonucleoside reductase inhibitor that augments the incorporation of decitabine into negative-sense viral DNA. When administered in tandem by Clouser and colleagues, decitabine and gemcitabine effected a 73% decrease in HIV infectivity. Clouser et al. reported that the application of the two drugs to HIV infected cells in vitro led to shifts in the HIV mutation spectrum secondary to increased rates of mutagenesis [29]. Importantly, the observed decrease in HIV infectivity could be attributed to error catastrophe following lethal mutagenesis by decitabine and gemcitabine and not the non-viability of affected viral DNA, because no decline in the rate of viral DNA synthesis was observed [28].
Combination decitabine and gemcitabine therapy for the treatment of HIV infection has recently performed favorably in preclinical evaluations. Both drugs were demonstrated to be non-toxic at therapeutic doses and successfully inhibited HIV infection in a murine AIDS model [30,31].
KP1461/KP1212
KP1461 is the prodrug of KP1212, a deoxycytidine nucleoside analog that indices A-to-G and G-to-A nucleoside transversion mutations when incorporated into viral DNA by a mechanism analogous to that of 5-AZC and decitabine. In phase II clinical trials, KP1212 reduced HIV titers to undetectable levels in cell culture after 9-24 rounds of viral replication. However, this reduction in HIV titers failed to persist, and all enrolled patients were seropositive for HIV by the end of the 125 day study period [32]. A 2014 study of KP1212 by Li and colleagues used advanced spectroscopic methods - including variable temperature NMR and 2D infrared spectroscopy - to further elucidate the mechanism of action of KP1212. Enol-keto tautaumerization, with enolic forms predominating, of KP1212 was found to influence the extent of A-to-G and G-to-A transversion mutations induced by the drug. This recent study may provide crucial insight into the mechanistic challenges faced by exogenous lethal mutagens and enable the translation of KP1212 into further clinical trials [33].
Future Prospects for HIV Therapeutics Based on Lethal Mutagenesis
The lethal mutagenesis of HIV in infected hosts by novel therapeutics offers an exciting and important advance towards the attainment of a safe and effective HIV cure.