Quorum sensing in vibrio cholerae: Difference between revisions
No edit summary |
|||
(16 intermediate revisions by one other user not shown) | |||
Line 1: | Line 1: | ||
{{Uncurated}} | |||
==Introduction== | ==Introduction== | ||
<br>By Stephanie Penix <br> | <br>By Stephanie Penix <br> | ||
<br> | [[Image: Vcholerae scanning.jpeg|thumb|300px|right|Figure 1: Scanning microscopy image of <i> Vibrio cholerae. </i> Retrieved from http://parasites.czu.cz/food/parasite.php?idParasite=61]] | ||
<br> Cholera is a severe diarrheal disease with ancient origins, with Sanskrit accounts of the disease from the Indian subcontinent dating to the fifth century B.C. (1). In the early 1850s, the British physician John Snow was the first to suggest that cholera was a communicable disease that was spread through a drinking supply contaminated with infected stools. The bacterium responsible for cholera was isolated in pure culture by Robert Koch in 1850. Today it is estimated to infect three to five million people annually, with cases being hugely under-reported. Although it has largely been eradicated from industrialized nations, cases worldwide have continued to grow since 2005 (CDC)(2). Cholera is endemic in 50 nations around the world (1). Outbreaks most commonly occur in Africa, Southeast Asia, and Haiti (CDC). Floods and other natural disasters often contribute to these outbreaks. | |||
The pathogenic agent of cholera is <i> Vibrio cholerae </i>, a Gram-negative, curved-rod shaped bacterium (Figure 1). There are many serogroups in this species; however, only two, 01 and 0139, are pathogenic (1). <i> V. cholerae </i> is able to alternate between living in the environment and within a host. In the environment, the bacterium is found in marine ecosystems and estuaries. <i> V. cholerae </i> is often found living symbiotically with zooplankton and shellfish, using the chitin found in their exoskeletons as a carbon and nitrogen source. | |||
As it is caused by this aquatic pathogen, cholera is most commonly spread by water supplies contaminated with stools infected with <i> V. cholerae </i>. It can be difficult to detect the presence of <i> V. cholerae </i> in water sources, due to the bacterium’s ability to aggregate into of conditionally viable environmental cells (CVECs) (3). CVECs form in infected stools and are then dispersed into water supplies. <i> V. cholerae </i> also forms biofilms upon colonization on their copepod symbionts. The formation of these CVECs is postulated to be evolutionarily beneficial because it could help <i> V. cholerae </i>persist in the environment as well as minimize the energetic cost of osmotic stress. CVECs are challenging to culture using standard techniques. However, CVECs have an extremely high potential of infectivity. While in the environment, they are in a partially dormant state but can reactivate upon entering a host. The biofilm can then disperse, leading to a burst of infectivity. | |||
The infectious dose of <i> V. cholerae </i> is between 10^5 −10^8 organisms for humans (1). However, other medical conditions such as co-infection with parasites and Vitamin A deficiency increase susceptibility to cholera infection. Most of the infecting microbes are killed by stomach acid. However, those that survive travel to the small intestine where they begin to produce cholera toxin (CT), which is the main virulence factor of <i> V. cholerae </i>. The B subunit of CT binds to the outside of the host cells, allowing the A sub-unit to enter the cell. Once inside, it activated adenyl cyclase, causing the concentration of cyclic-AMP to increase. These heightened cAMP levels cause the diarrhea that is characteristic of cholera. Regulated in parallel to CT are pilus genes, which allow effective colonization of the digestive tract. | |||
Prevention | Although the mechanism of cholera infection has been well-studied, it remains a problem, especially in developing countries. Prevention of cholera is contingent on the availability of clean water and the development of sanitation facilities (4). Health education about proper food hygiene is also important in reducing the transmission of the disease. Mass treatment of communities with antibiotics has been shown to have no benefit, and can actually be detrimental as it can lead to antibiotic resistance. There are two types of vaccines available for <i> V. cholerae </i>, a parenteral vaccine and an oral vaccine. The WHO does not encourage use of the parenteral vaccine, due to high potential of deleterious side effects and relatively low efficacy. The oral vaccine is available for individuals travelling to countries where cholera is endemic, and has recently started to be used during outbreaks of the disease. Left untreated, cholera can have a fatality rate of over fifty percent (1). However, effective therapy, which involves aggressive fluid and electrolyte replacement, can lower this mortality rate to less than one percent. | ||
<br> | <br> | ||
==Quorum Sensing and Cell Density== | ==Quorum Sensing and Cell Density== | ||
[[Image:Quorum Sensing in Vibrio cholerae.jpeg|thumb|300px|right|Regulation of biofilm formation in | [[Image:Quorum Sensing in Vibrio cholerae.jpeg|thumb|300px|right|Figure 2. Regulation of biofilm formation in <i> V. cholerae </i> at high and low cell densities. Retrieved from http://jb.asm.org/content/190/7/2527.full]] | ||
Quorum sensing is a type of bacterial communication that occurs via the secretion of autoinducer molecules (5). Bacteria can detect the presence of autoinducers produced by members of the same species. At a critical level of environmental autoinducers, gene expression in the members of the colony changes and the behavior of the colony as a whole is altered. | Quorum sensing is a type of bacterial communication that occurs via the secretion of autoinducer molecules (5). Bacteria can detect the presence of autoinducers produced by members of the same species. At a critical level of environmental autoinducers, gene expression in the members of the colony changes and the behavior of the colony as a whole is altered. <i> V. cholerae </i> must contend with two distinct environmental conditions: free-floating in the environment and attachment to a host (6). Consequently, the bacterium must be able to effectively transition between these two phases through differential gene expression patterns. This transition is facilitated through both quorum sensing and a second regulatory system, 3′,5′-cyclic diguanylic acid (c-di-GMP) signaling. | ||
Quorum sensing in <i> V. cholerae </i> is governed by parallel phosphorylation cascades. The first system uses the autoinducer AI-2, a furanosyl borate diester, whose presence in the cell is detected by LuxPQ (7). AI-2 is found in many other families of bacteria besides <i> Vibrio </i> (8). The autoinducer used in the second system is cholera autoinducer-1 (CAI-1), a hydroxylated alkyl ketone, which is detected by CqsS (5). Unlike AI-2, CAI-1 is unique to the <i> Vibrio </i> family (8). At low concentrations of autoinducers, indicating low cell density, A1 molecules act as kinases, transferring phosphate groups to the response regulator LuxO. The phosphorylated version of LuxO, in conjunction with a second protein, LuxP, activates the genes encoding Qrr small regulatory mRNA molecules (9). These regulatory mRNA molecules destabilize the mRNA encoding a major quorum sensing transcription factor, HapR. This destabilization results in downregulation of quorum sensing, so that the low-cell density phenotype of biofilm formation can be expressed. It also results in the upregulation of the extracellular proteases HapA and PrtV (10). Figure 2 gives a summary of the biofilm formation pathway in <i> V. cholerae </i> and how it is affected by high and low cell density. | |||
When the concentration of autoinducers increases, these molecules bind their cognate receptors, resulting in a conformational change that confers phosphatase activity. The receptors then begin to remove the phosphate groups from LuxO (5). As a result, the production of the small regulatory mRNAs is lessened, and the HapR mRNA is stabilized and can be translated to protein. The expression of HapR signals to the bacteria that it is in a high-cell density environment, and results in downregulation of both biofilm and virulence genes, which aids in the dispersal of the bacteria. | When the concentration of autoinducers increases, these molecules bind their cognate receptors, resulting in a conformational change that confers phosphatase activity. The receptors then begin to remove the phosphate groups from LuxO (5). As a result, the production of the small regulatory mRNAs is lessened, and the HapR mRNA is stabilized and can be translated to protein. The expression of HapR signals to the bacteria that it is in a high-cell density environment, and results in downregulation of both biofilm and virulence genes, which aids in the dispersal of the bacteria. | ||
The biofilm phenotype is activated by the regulator c-di-GMP, a second-messenger molecule that switches the cell from a motile to a sessile state (5). High levels of c-di-GMP promote biofilm formation and repress virulence and motility (6). The molecule is generated when two GTP molecules cyclize with the loss of two phosphate groups, a process carried out by proteins with GGDEF motifs such as QrgB. HapR has an effect on this cycle because it represses the expression of GGDEF proteins. Thus, when cell density HapR concentrations are low, more c-di-GMP is produced. The molecule c-di-GMP acts by inducing the biofilm activator vpsT. HapR exerts a second type of control on biofilms formation by binding to vpsT, inhibiting the expression of biofilm genes. A second type of regulation that affects c-di-GMP levels is the protein VieA, controlled by the promoter V1086, which contains an EAL domain that acts as a c-di-GMP phosphodiesterase, thereby degrading the molecule and reducing its overall cellular concentration. As a result, exopolysaccharide genes, which are important in the formation of biofilms, are repressed (11). The role of c-di-GMP was demonstrated in experiments where abnormally high concentrations of the molecule were expressed at high cell density. In this high-density system, the biofilm phenotype characteristic of low cell density was observed. | The biofilm phenotype is activated by the regulator c-di-GMP, a second-messenger molecule that switches the cell from a motile to a sessile state (5). High levels of c-di-GMP promote biofilm formation and repress virulence and motility (6). The molecule is generated when two GTP molecules cyclize with the loss of two phosphate groups, a process carried out by proteins with GGDEF motifs such as QrgB. HapR has an effect on this cycle because it represses the expression of GGDEF proteins. Thus, when cell density HapR concentrations are low, more c-di-GMP is produced. The molecule c-di-GMP acts by inducing the biofilm activator vpsT. HapR exerts a second type of control on biofilms formation by binding to vpsT, inhibiting the expression of biofilm genes. A second type of regulation that affects c-di-GMP levels is the protein VieA, controlled by the promoter V1086, which contains an EAL domain that acts as a c-di-GMP phosphodiesterase, thereby degrading the molecule and reducing its overall cellular concentration. As a result, exopolysaccharide genes, which are important in the formation of biofilms, are repressed (11). The role of c-di-GMP was demonstrated in experiments where abnormally high concentrations of the molecule were expressed at high cell density. In this high-density system, the biofilm phenotype characteristic of low cell density was observed. | ||
<br> | <br> | ||
<br> | <br> | ||
==Competence== | ==Competence== | ||
Competence, which is the ability of a microbe to take up DNA from the external environment, is a process that occurs in V. cholerae. The DNA absorbed can then undergo transformation into the genome through recombination. Being naturally competent allows the possibility of horizontal gene transfer (HGT). The presence of large genomic islands in the genome of V. cholerae indicates that HGT has occurred throughout its evolutionary history (8). Transformation could allow for the acquisition of virulence genes by nonpathogenic strains of V. cholerae. | Competence, which is the ability of a microbe to take up DNA from the external environment, is a process that occurs in <i> V. cholerae </i>. The DNA absorbed can then undergo transformation into the genome through recombination. Being naturally competent allows the possibility of horizontal gene transfer (HGT). The presence of large genomic islands in the genome of <i> V. cholerae </i> indicates that HGT has occurred throughout its evolutionary history (8). Transformation could allow for the acquisition of virulence genes by nonpathogenic strains of <i> V. cholerae </i>. | ||
Chitin, found in the exoskeletons on which V. cholerae live symbiotically, is required for the induction of competence and subsequent transformation in V. cholerae (12). The detection of chitin and subsequent alteration of gene expression to allow for competence and transformation is regulated by the protein TFox. However, other factors, such as high concentrations of cyclic-AMP in the cell, are required for transformation to occur. | Chitin, found in the exoskeletons on which <i> V. cholerae </i> live symbiotically, is required for the induction of competence and subsequent transformation in <i> V. cholerae </i> (12). The detection of chitin and subsequent alteration of gene expression to allow for competence and transformation is regulated by the protein TFox. However, other factors, such as high concentrations of cyclic-AMP in the cell, are required for transformation to occur. | ||
Quorum sensing also has an effect on whether DNA uptake will occur. Both of the autoinducers that V. cholerae produces contribute to the expression of comEA. However, CAI-1 is more important for this process, with AI-2 playing a less significant role (8). The quorum sensing transcription factor HapR is also required for transformation (12). While quorum sensing does not regulate all of the genes involved in transformation, it does regulate two: comEA and comEC. ComEA encodes a DNA-binding protein located in the perisplasm that activates competence-involved genes. ComEC is directly involved in taking up DNA from the external environment. | Quorum sensing also has an effect on whether DNA uptake will occur. Both of the autoinducers that <i> V. cholerae </i> produces contribute to the expression of comEA. However, CAI-1 is more important for this process, with AI-2 playing a less significant role (8). The quorum sensing transcription factor HapR is also required for transformation (12). While quorum sensing does not regulate all of the genes involved in transformation, it does regulate two: comEA and comEC. ComEA encodes a DNA-binding protein located in the perisplasm that activates competence-involved genes. ComEC is directly involved in taking up DNA from the external environment. | ||
Quorum sensing also acts to regulate the nuclease dns (12). Under conditions of low cell density, low concentrations of HapR are present and dns is transcribed and translated to protein. This protein is excreted from the cell and acts to degrade free-floating DNA in the environment. Thus, there is not DNA available to recombine into the genome of V. cholerae. The reverse process occurs at high cell density when high concentrations of HapR are present. Less of the nuclease is produced, and as a result, there is a possibility for surrounding DNA to be taken up. | Quorum sensing also acts to regulate the nuclease dns (12). Under conditions of low cell density, low concentrations of HapR are present and dns is transcribed and translated to protein. This protein is excreted from the cell and acts to degrade free-floating DNA in the environment. Thus, there is not DNA available to recombine into the genome of <i> V. cholerae </i>. The reverse process occurs at high cell density when high concentrations of HapR are present. Less of the nuclease is produced, and as a result, there is a possibility for surrounding DNA to be taken up. | ||
The induction of competence does not happen equally to all V. cholerae cells in a population (12). This disparity is postulated to exist due to unequal distribution of autoinducers in the environment, as well as unequal distribution of the chitin derivatives required to induce competence genes. | The induction of competence does not happen equally to all <i> V. cholerae </i> cells in a population (12). This disparity is postulated to exist due to unequal distribution of autoinducers in the environment, as well as unequal distribution of the chitin derivatives required to induce competence genes. | ||
<br> | <br> | ||
<br> | <br> | ||
Line 35: | Line 41: | ||
==Quorum Sensing Cheaters== | ==Quorum Sensing Cheaters== | ||
<br> | <br> | ||
[[Image: QS cheating.png|thumb|300px|right|When V. cholerae are grown on casein protein, the prevalence of QS-deficiency increases, while this increase is not observed when glucose is the nutrient source. Retrieved from http://aem.asm.org/content/early/2015/03/23/AEM.00586-15.abstract]] | [[Image: QS cheating.png|thumb|300px|right|Figure 3. When <i> V. cholerae </i> are grown on casein protein, the prevalence of QS-deficiency increases, while this increase is not observed when glucose is the nutrient source. Retrieved from http://aem.asm.org/content/early/2015/03/23/AEM.00586-15.abstract]] | ||
One emerging area of study in quorum sensing is the significance of the role of signaling-deficient bacteria. Quorum sensing depends on individual bacteria expending energy to produce signals and proteins that other nearby individuals are also producing. Thus, it is a pathway that is susceptible to the emergence of social cheaters who capitalize on the shared resources but do not produce any themselves. Genetic analysis of | One emerging area of study in quorum sensing is the significance of the role of signaling-deficient bacteria. Quorum sensing depends on individual bacteria expending energy to produce signals and proteins that other nearby individuals are also producing. Thus, it is a pathway that is susceptible to the emergence of social cheaters who capitalize on the shared resources but do not produce any themselves. Genetic analysis of <i> V. cholerae </i> reveals that deficiencies in quorum sensing are coming in <i> V. cholerae </i>; however, it is less common among pandemic strains (13). In fact, the first genome of <i> V. cholerae </i> to be sequenced, from the strain N16961, was shown to be deficient in quorum sensing (12). The majority of these deficiencies stem from mutations in the genes encoding hapR (10). The evolutionary viability of QS cheaters is reinforced by the fact that clinical and environmental isolates show a variety of mutations in hapR, suggesting that these mutations often occur de novo and are not just transferred between populations. Despite this evolutionary benefit to hapR mutations, there is also a downside in that deficiencies in this gene are associated with reduced competence of <i> V. cholerae </i>. Thus, a fitness trade-off is likely to exist in the acquisition of hapR mutations because these mutations could result in lower ability to take up genetic material from the external environment. | ||
Populations of V. cholerae with a high prevalence of QS mutants produce similar levels of signaling molecules to populations without these mutants, implying that the QS-mutants are signaling other individuals to produce these molecules (10). A relatively low prevalence of organisms capable of producing quorum sensing molecules appears to be sufficient to sustain the biofilm. However, it is thought that the presence of these QS-deficient bacteria could lower the antibiotic resistance of the biofilm and decrease its overall productivity. | Populations of <i> V. cholerae </i> with a high prevalence of QS mutants produce similar levels of signaling molecules to populations without these mutants, implying that the QS-mutants are signaling other individuals to produce these molecules (10). A relatively low prevalence of organisms capable of producing quorum sensing molecules appears to be sufficient to sustain the biofilm. However, it is thought that the presence of these QS-deficient bacteria could lower the antibiotic resistance of the biofilm and decrease its overall productivity. | ||
Specific environmental conditions are conducive to the emergence of QS mutants, while other sets of conditions do not appear to cause these developments (10). Under conditions of LB, simulated seawater, and pondwater, prevalence of hapR mutations did not increase. However, being a QS mutant is advantageous to V. cholerae when in the presence of wild type organisms in a biofilm. The nutrient availability also plays a role. When V. cholerae are cultured with glucose as a nutrient source, the prevalence of QS mutations does not increase. However, when casein protein is the only nutrient source, QS-mutant organisms do increase in proportion to the entire population. Interestingly, some mutations observed were identical to those previously sequenced in clinical and environmental samples. Under conditions in which hapR mutants become more common, the total number of cells did not significantly change, indicating that these mutations were not causing large problems for the biofilm as a whole. In addition, quorum sensing mutations were favored specifically under biofilm conditions and not during planktonic growth. This observation is clinically relevant considering that V. cholerae infecting humans is likely to be growing on surfaces and not free-floating. | Specific environmental conditions are conducive to the emergence of QS mutants, while other sets of conditions do not appear to cause these developments (10). Under conditions of LB, simulated seawater, and pondwater, prevalence of hapR mutations did not increase. However, being a QS mutant is advantageous to <i> V. cholerae </i> when in the presence of wild type organisms in a biofilm. The nutrient availability also plays a role. When <i> V. cholerae </i> are cultured with glucose as a nutrient source, the prevalence of QS mutations does not increase. However, when casein protein is the only nutrient source, QS-mutant organisms do increase in proportion to the entire population, as demonstrated in Figure 3. Interestingly, some mutations observed were identical to those previously sequenced in clinical and environmental samples. Under conditions in which hapR mutants become more common, the total number of cells did not significantly change, indicating that these mutations were not causing large problems for the biofilm as a whole. In addition, quorum sensing mutations were favored specifically under biofilm conditions and not during planktonic growth. This observation is clinically relevant considering that <i> V. cholerae </i> infecting humans is likely to be growing on surfaces and not free-floating. | ||
One mechanism that could explain the advantage of QS deficiency for the individual microbe is that is lowers the expression of extracellular proteases (10). In V. cholerae, HapR activates two proteases, HapA and PrtV, in the process of quorum sensing. A strain with both hapR and PrtV deleted was unable to grown in casein-rich medium. When only one of the proteases was deleted, growth did occur. However, no QS-mutants developed over extended growth of the single protease-knockout strains. This result suggests that the metabolic cost avoided by lacking one of the proteases counterbalances the potential energetic benefit of incurring a QS mutation. | One mechanism that could explain the advantage of QS deficiency for the individual microbe is that is lowers the expression of extracellular proteases (10). In <i> V. cholerae </i>, HapR activates two proteases, HapA and PrtV, in the process of quorum sensing. A strain with both hapR and PrtV deleted was unable to grown in casein-rich medium. When only one of the proteases was deleted, growth did occur. However, no QS-mutants developed over extended growth of the single protease-knockout strains. This result suggests that the metabolic cost avoided by lacking one of the proteases counterbalances the potential energetic benefit of incurring a QS mutation. | ||
Additionally, there was no difference in the amount of the QS autoinducers AI1 and CAI-1 between colonies with a high presence of QS mutations and those without (10). This lack of difference can be explained by the fact that HapR, the quorum sensing master regulator, does not have an effect on autoinducer levels and is not required for their production. Thus, organisms deficient in HapR would be producing equivalent levels of autoinducers to the wild type. | Additionally, there was no difference in the amount of the QS autoinducers AI1 and CAI-1 between colonies with a high presence of QS mutations and those without (10). This lack of difference can be explained by the fact that HapR, the quorum sensing master regulator, does not have an effect on autoinducer levels and is not required for their production. Thus, organisms deficient in HapR would be producing equivalent levels of autoinducers to the wild type. | ||
Social cheating of V. cholerae could be especially relevant in the context of algae blooms (10). Under these conditions, there are high concentrations of dissolved organic matter in the form of proteins and carbohydrates. In addition, the protein found in the fecal matter of V. cholerae hosts could be conducive to the emergence of social cheaters. | Social cheating of <i> V. cholerae </i> could be especially relevant in the context of algae blooms (10). Under these conditions, there are high concentrations of dissolved organic matter in the form of proteins and carbohydrates. In addition, the protein found in the fecal matter of <i> V. cholerae </i> hosts could be conducive to the emergence of social cheaters. | ||
==Therapeutic Opportunities== | ==Therapeutic Opportunities== | ||
[[Image:CT_mouse.png|thumb|300px|right|Presence of cholera toxin (red color) in infant mouse intestines. Prior to infection with V. cholerae, mice were treated with A) nothing, B) E coli Nissle or C) E coli transformed to express V. cholerae cqsA. which encodes the enzyme CAI-1. Group D) had no pretreatment or infection. Retrieved from http://www.ncbi.nlm.nih.gov/pmc/articles/PMC2895089/]] | [[Image:CT_mouse.png|thumb|300px|right|Presence of cholera toxin (red color) in infant mouse intestines. Prior to infection with <i> V. cholerae </i>, mice were treated with A) nothing, B) <i> E. coli </i> Nissle or C) <i> E. coli </i> transformed to express <i> V. cholerae </i> cqsA. which encodes the enzyme CAI-1. Group D) had no pretreatment or infection. Retrieved from http://www.ncbi.nlm.nih.gov/pmc/articles/PMC2895089/]] | ||
<br> The possibility of antibiotic resistance is a huge concern in the development of new anti-microbial agents. One way to minimize the rise of resistant microbes is to design drugs that alter the behavior of bacteria instead of disrupting cellular functioning to kill the cell (14). The idea behind this approach is that it results in less selective pressure, hypothetically lowering the potential for microbes to evolve to be resistant. Quorum sensing is a process that could theoretically be disrupted in this manner, altering the pathogenesis of a bacterium but not necessarily killing it. | |||
When V. cholerae initially infects a host, there are very low levels of autoinducers present, so the genes for both biofilm and virulence are activated (14). As the bacterial density in the host rises, V. cholerae transitions out of the biofilm phase and becomes less virulent. Proteases that are produced in response to the autoinducer CAI1 cause the bacterium to detach from the walls of the intestine, so it can pass from the host’s system. One possibility to fight cholera infections, then, is to introduce CAI1 into the host which could trigger the deactivation of biofilm and virulence phenotypes. | When <i> V. cholerae </i> initially infects a host, there are very low levels of autoinducers present, so the genes for both biofilm and virulence are activated (14). As the bacterial density in the host rises, V. cholerae transitions out of the biofilm phase and becomes less virulent. Proteases that are produced in response to the autoinducer CAI1 cause the bacterium to detach from the walls of the intestine, so it can pass from the host’s system. One possibility to fight cholera infections, then, is to introduce CAI1 into the host which could trigger the deactivation of biofilm and virulence phenotypes. | ||
One approach to introduce autoinducers into the host is through genetically engineered commensal bacteria (15). Nissle, which is a model commensal strain of E coli, has been transformed to express cqSA, the gene encoding the enzyme that catalyzes the synthesis of CAI-1. It was linked to the promoter fli-C, which is constitutively expressed. Mice that were fed these transformed bacteria and subsequently infected with V. cholerae showed much higher survival rates in a dose-dependent manner. The administration of the commensal bacteria lowered the accumulation of cholera toxin (CT) as well as lowered the extent of V. cholerae binding to the intestine. The duration of the treatment was extremely important for the potential of this system as a prophylactic, as | One approach to introduce autoinducers into the host is through genetically engineered commensal bacteria (15). Nissle, which is a model commensal strain of <i> E. coli </i>, has been transformed to express cqSA, the gene encoding the enzyme that catalyzes the synthesis of CAI-1. It was linked to the promoter fli-C, which is constitutively expressed. Mice that were fed these transformed bacteria and subsequently infected with <i> V. cholerae </i> showed much higher survival rates in a dose-dependent manner. The researchers quantified the number of colony-forming units (CFUs)of <i> V. cholerae </i> present in the intestines of mice that were infected with the cqSA-producing bacteria and those that were not, and found that <i> V. cholerae </i> were less likely to be present in the former group. The administration of the commensal bacteria lowered the accumulation of cholera toxin (CT) as well as lowered the extent of <i> V. cholerae </i> binding to the intestine. Figure 4 shows the lower levels of cholera toxin that accumulate in mouse intestines when the mice are co-infected with <i> E coli </i> expressing <i> V. cholerae </i> cqSA. The duration of the treatment was extremely important for the potential of this system as a prophylactic, as pre-treatment at 8 hours was much more effective than at 4 hours. A similar prophylactic approach could be used as a preventive measure where the possibility of cholera epidemic is high, such as areas that have recently experienced disruption of infrastructure due to a natural disaster. A limitation to this approach is that adult mice are not susceptible to lasting infection by <i> V. cholerae </i>, so the window of study is short. | ||
An oral therapy | An alternative to infection with live transformed bacteria would be an oral nanoparticle therapy where CAI-1 is covered in a soluble coating to improve its delivery. One difficulty in designing an oral therapy for CAI-1 is that it is a large, hydrophobic molecule (14). As a result, it would be challenging to deliver it at a high enough concentration to have a therapeutic effect. To address this problem, nanocarriers have been considered as an alternative. This approach involves coating the hydrophobic molecule with polyethylene glycol (PEG). Nanoparticles improve the kinetics of the drug, allowing it to be better solubilized. It also aids its passage through mucus layers. Flash Nano Precipitation was used to make the CAI-1 nanoparticles, a protocol which has been employed in other medicinal studies to improve the kinetics of hydrophobic drugs. In order to effectively target <i> V. cholerae </i>, a nanoparticle would have to be impermeable to acid so it could pass through the stomach to the small intestine without being degraded. The bile micelles in the small intestine then dissolve the CAI1 particles, releasing the contents, while also facilitating transport across the mucus layer. | ||
In order to test the effectiveness of this potential therapy, the creators of the nanoparticle system used a reporter strain of V. cholerae that cannot produce its own CAI-1, but does have a luciferase insert that is activated in the presence of external CAI-1. This pathway, which results in bioluminescence, is the same cascade for biofilm and virulence gene expression. However, the effect is reversed, because bioluminescence is activated by CAI-1 while the biofilm and virulence phenotypes are repressed. These assays demonstrated that there were higher levels of bioluminescence produced in the presence of nanoparticle CAI-1 than free CAI-1. Thus, nanoparticles are a promising avenue for delivery of autoinducers as a therapeutic. | In order to test the effectiveness of this potential therapy, the creators of the nanoparticle system used a reporter strain of <i> V. cholerae </i> that cannot produce its own CAI-1, but does have a luciferase insert that is activated in the presence of external CAI-1. This pathway, which results in bioluminescence, is the same cascade for biofilm and virulence gene expression. However, the effect is reversed, because bioluminescence is activated by CAI-1 while the biofilm and virulence phenotypes are repressed. These assays demonstrated that there were higher levels of bioluminescence produced in the presence of nanoparticle CAI-1 than free CAI-1. Thus, nanoparticles are a promising avenue for delivery of autoinducers as a therapeutic. | ||
<br> | <br> | ||
Line 65: | Line 71: | ||
==References== | ==References== | ||
[1] [http://www. | [1] [http://www.thelancet.com/journals/lancet/article/PIIS0140-6736%2812%2960436-X/abstract J. B. Harris, R. C. LaRocque, F. Qadri, E. T. Ryan, S. B. Calderwood, Cholera. Lancet 379, 2466-2476 (2012).] | ||
[2] [http://www.cdc.gov/cholera/index.html | [2] [http://www.cdc.gov/cholera/index.html CDC - Topic Title - Healthy Water. (2015).] | ||
[3] [http://www.ncbi.nlm.nih.gov/pmc/articles/PMC1458881 | [3] [http://www.ncbi.nlm.nih.gov/pmc/articles/PMC1458881 S. M. Faruque et al., Transmissibility of cholera: in vivo-formed biofilms and their relationship to infectivity and persistence in the environment. Proc Natl Acad Sci U S A 103, 6350-6355 (2006).] | ||
[4] [http://www.who.int/cholera/technical/prevention/control/en | [4] [http://www.who.int/cholera/technical/prevention/control/en WHO | Prevention and control of cholera outbreaks: WHO policy and recommendations in WHO. (World Health Organization, 2011).] | ||
[5] [http://onlinelibrary.wiley.com/doi/10.1046/j.1365-2958.2003.03688.x/abstract;jsessionid=4753624BD55F7DE47F707CCC68C18EF1.f02t02 | [5] [http://onlinelibrary.wiley.com/doi/10.1046/j.1365-2958.2003.03688.x/abstract;jsessionid=4753624BD55F7DE47F707CCC68C18EF1.f02t02 B. K. Hammer, P. U. Department of Molecular Biology, Princeton, New Jersey 08544–1014, USA., B. L. Bassler, Quorum sensing controls biofilm formation in Vibrio cholerae. Molecular Microbiology 50, 101-104 (2003).] | ||
[6] [http://jb.asm.org/content/190/7/2527.long | [6] [http://jb.asm.org/content/190/7/2527.long C. M. Waters, W. Lu, J. D. Rabinowitz, B. L. Bassler, Quorum Sensing Controls Biofilm Formation in <i> Vibrio cholerae </i> through Modulation of Cyclic Di-GMP Levels and Repression of vpsT. (2008).] | ||
[7] [http://jb.asm.org/content/194/17/4485.long | [7] [http://jb.asm.org/content/194/17/4485.long D. Srivastava, C. M. Waters, A tangled web: regulatory connections between quorum sensing and cyclic Di-GMP. J Bacteriol 194, 4485-4493 (2012).] | ||
[8] [http://femsle.oxfordjournals.org/cgi/pmidlookup?view=long&pmid=21658103 | [8] [http://femsle.oxfordjournals.org/cgi/pmidlookup?view=long&pmid=21658103 E. S. Antonova, B. K. Hammer, Quorum-sensing autoinducer molecules produced by members of a multispecies biofilm promote horizontal gene transfer to <i> Vibrio cholerae </i>. FEMS Microbiol Lett 322, 68-76 (2011).] | ||
[9] [http://www.sciencedirect.com/science/article/pii/S0092867404005732 | [9] [http://www.sciencedirect.com/science/article/pii/S0092867404005732 D. H. Lenz et al., The small RNA chaperone Hfq and multiple small RNAs control quorum sensing in <i> Vibrio harvey </i> and <i> Vibrio cholerae </i>. Cell. (United States, 2004), vol. 118, pp. 69-82.] | ||
[10] [http://aem.asm.org/content/early/2015/03/23/AEM.00586-15.long | [10] [http://aem.asm.org/content/early/2015/03/23/AEM.00586-15.long D. S. Katzianer, H. Wang, R. M. Carey, J. Zhu, Quorum non-sensing: social cheating and deception in <i> Vibrio cholerae </i>. Appl Environ Microbiol, (2015).] | ||
[11] [http://www.ncbi.nlm.nih.gov/pmc/articles/PMC2790424/ | [11] [http://www.ncbi.nlm.nih.gov/pmc/articles/PMC2790424/ A. D. Tischler, A. Camilli, Cyclic diguanylate (c-di-GMP) regulates <i> Vibrio cholerae </i> biofilm formation. Mol Microbiol. (2004 Blackwell Publishing Ltd, England, 2004), vol. 53, pp. 857-869.] | ||
[12] [http://www.ncbi.nlm.nih.gov/pmc/articles/PMC3575429/ | [12] [http://www.ncbi.nlm.nih.gov/pmc/articles/PMC3575429/ M. Blokesch, A quorum sensing-mediated switch contributes to natural transformation of <i> Vibrio cholerae </i> Mob Genet Elements. (2012), vol. 2, pp. 224-227.] | ||
[13] [http://www.ncbi.nlm.nih.gov/pmc/articles/PMC3107014/ | [13] [http://www.ncbi.nlm.nih.gov/pmc/articles/PMC3107014/ Y. Wang et al., The Prevalence of Functional Quorum-Sensing Systems in Recently Emerged <i> Vibrio cholerae </i> Toxigenic Strains. Environ Microbiol Rep 3, 218-222 (2011).] | ||
[14] [http://pubs.acs.org/doi/abs/10.1021/acs.nanolett.5b00151 | [14] [http://pubs.acs.org/doi/abs/10.1021/acs.nanolett.5b00151 H. D. Lu et al., Modulating <i> Vibrio cholerae </i> Quorum-Sensing-Controlled Communication Using Autoinducer-Loaded Nanoparticles. Nano Lett 15, 2235-2241 (2015).] | ||
[15] [http://www.ncbi.nlm.nih.gov/pmc/articles/PMC2895089/ | [15] [http://www.ncbi.nlm.nih.gov/pmc/articles/PMC2895089/ F. Duan, J. C. March, Engineered bacterial communication prevents <i> Vibrio cholerae </i> virulence in an infant mouse model. Proc Natl Acad Sci U S A 107, 11260-11264 (2010).] | ||
<br><br>Authored for [http://biology.kenyon.edu/courses/biol238/biol238syl15.html BIOL 238 Microbiology], taught by [mailto:slonczewski@kenyon.edu Joan Slonczewski], 2015, [http://www.kenyon.edu/index.xml Kenyon College]. | <br><br>Authored for [http://biology.kenyon.edu/courses/biol238/biol238syl15.html BIOL 238 Microbiology], taught by [mailto:slonczewski@kenyon.edu Joan Slonczewski], 2015, [http://www.kenyon.edu/index.xml Kenyon College]. |
Latest revision as of 20:27, 29 September 2015
Introduction
By Stephanie Penix

Cholera is a severe diarrheal disease with ancient origins, with Sanskrit accounts of the disease from the Indian subcontinent dating to the fifth century B.C. (1). In the early 1850s, the British physician John Snow was the first to suggest that cholera was a communicable disease that was spread through a drinking supply contaminated with infected stools. The bacterium responsible for cholera was isolated in pure culture by Robert Koch in 1850. Today it is estimated to infect three to five million people annually, with cases being hugely under-reported. Although it has largely been eradicated from industrialized nations, cases worldwide have continued to grow since 2005 (CDC)(2). Cholera is endemic in 50 nations around the world (1). Outbreaks most commonly occur in Africa, Southeast Asia, and Haiti (CDC). Floods and other natural disasters often contribute to these outbreaks.
The pathogenic agent of cholera is Vibrio cholerae , a Gram-negative, curved-rod shaped bacterium (Figure 1). There are many serogroups in this species; however, only two, 01 and 0139, are pathogenic (1). V. cholerae is able to alternate between living in the environment and within a host. In the environment, the bacterium is found in marine ecosystems and estuaries. V. cholerae is often found living symbiotically with zooplankton and shellfish, using the chitin found in their exoskeletons as a carbon and nitrogen source.
As it is caused by this aquatic pathogen, cholera is most commonly spread by water supplies contaminated with stools infected with V. cholerae . It can be difficult to detect the presence of V. cholerae in water sources, due to the bacterium’s ability to aggregate into of conditionally viable environmental cells (CVECs) (3). CVECs form in infected stools and are then dispersed into water supplies. V. cholerae also forms biofilms upon colonization on their copepod symbionts. The formation of these CVECs is postulated to be evolutionarily beneficial because it could help V. cholerae persist in the environment as well as minimize the energetic cost of osmotic stress. CVECs are challenging to culture using standard techniques. However, CVECs have an extremely high potential of infectivity. While in the environment, they are in a partially dormant state but can reactivate upon entering a host. The biofilm can then disperse, leading to a burst of infectivity.
The infectious dose of V. cholerae is between 10^5 −10^8 organisms for humans (1). However, other medical conditions such as co-infection with parasites and Vitamin A deficiency increase susceptibility to cholera infection. Most of the infecting microbes are killed by stomach acid. However, those that survive travel to the small intestine where they begin to produce cholera toxin (CT), which is the main virulence factor of V. cholerae . The B subunit of CT binds to the outside of the host cells, allowing the A sub-unit to enter the cell. Once inside, it activated adenyl cyclase, causing the concentration of cyclic-AMP to increase. These heightened cAMP levels cause the diarrhea that is characteristic of cholera. Regulated in parallel to CT are pilus genes, which allow effective colonization of the digestive tract.
Although the mechanism of cholera infection has been well-studied, it remains a problem, especially in developing countries. Prevention of cholera is contingent on the availability of clean water and the development of sanitation facilities (4). Health education about proper food hygiene is also important in reducing the transmission of the disease. Mass treatment of communities with antibiotics has been shown to have no benefit, and can actually be detrimental as it can lead to antibiotic resistance. There are two types of vaccines available for V. cholerae , a parenteral vaccine and an oral vaccine. The WHO does not encourage use of the parenteral vaccine, due to high potential of deleterious side effects and relatively low efficacy. The oral vaccine is available for individuals travelling to countries where cholera is endemic, and has recently started to be used during outbreaks of the disease. Left untreated, cholera can have a fatality rate of over fifty percent (1). However, effective therapy, which involves aggressive fluid and electrolyte replacement, can lower this mortality rate to less than one percent.
Quorum Sensing and Cell Density
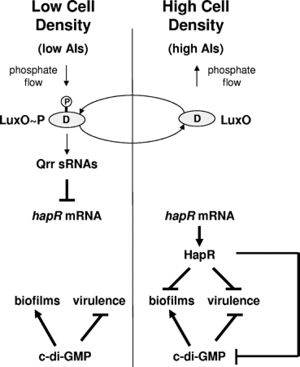
Quorum sensing is a type of bacterial communication that occurs via the secretion of autoinducer molecules (5). Bacteria can detect the presence of autoinducers produced by members of the same species. At a critical level of environmental autoinducers, gene expression in the members of the colony changes and the behavior of the colony as a whole is altered. V. cholerae must contend with two distinct environmental conditions: free-floating in the environment and attachment to a host (6). Consequently, the bacterium must be able to effectively transition between these two phases through differential gene expression patterns. This transition is facilitated through both quorum sensing and a second regulatory system, 3′,5′-cyclic diguanylic acid (c-di-GMP) signaling.
Quorum sensing in V. cholerae is governed by parallel phosphorylation cascades. The first system uses the autoinducer AI-2, a furanosyl borate diester, whose presence in the cell is detected by LuxPQ (7). AI-2 is found in many other families of bacteria besides Vibrio (8). The autoinducer used in the second system is cholera autoinducer-1 (CAI-1), a hydroxylated alkyl ketone, which is detected by CqsS (5). Unlike AI-2, CAI-1 is unique to the Vibrio family (8). At low concentrations of autoinducers, indicating low cell density, A1 molecules act as kinases, transferring phosphate groups to the response regulator LuxO. The phosphorylated version of LuxO, in conjunction with a second protein, LuxP, activates the genes encoding Qrr small regulatory mRNA molecules (9). These regulatory mRNA molecules destabilize the mRNA encoding a major quorum sensing transcription factor, HapR. This destabilization results in downregulation of quorum sensing, so that the low-cell density phenotype of biofilm formation can be expressed. It also results in the upregulation of the extracellular proteases HapA and PrtV (10). Figure 2 gives a summary of the biofilm formation pathway in V. cholerae and how it is affected by high and low cell density.
When the concentration of autoinducers increases, these molecules bind their cognate receptors, resulting in a conformational change that confers phosphatase activity. The receptors then begin to remove the phosphate groups from LuxO (5). As a result, the production of the small regulatory mRNAs is lessened, and the HapR mRNA is stabilized and can be translated to protein. The expression of HapR signals to the bacteria that it is in a high-cell density environment, and results in downregulation of both biofilm and virulence genes, which aids in the dispersal of the bacteria.
The biofilm phenotype is activated by the regulator c-di-GMP, a second-messenger molecule that switches the cell from a motile to a sessile state (5). High levels of c-di-GMP promote biofilm formation and repress virulence and motility (6). The molecule is generated when two GTP molecules cyclize with the loss of two phosphate groups, a process carried out by proteins with GGDEF motifs such as QrgB. HapR has an effect on this cycle because it represses the expression of GGDEF proteins. Thus, when cell density HapR concentrations are low, more c-di-GMP is produced. The molecule c-di-GMP acts by inducing the biofilm activator vpsT. HapR exerts a second type of control on biofilms formation by binding to vpsT, inhibiting the expression of biofilm genes. A second type of regulation that affects c-di-GMP levels is the protein VieA, controlled by the promoter V1086, which contains an EAL domain that acts as a c-di-GMP phosphodiesterase, thereby degrading the molecule and reducing its overall cellular concentration. As a result, exopolysaccharide genes, which are important in the formation of biofilms, are repressed (11). The role of c-di-GMP was demonstrated in experiments where abnormally high concentrations of the molecule were expressed at high cell density. In this high-density system, the biofilm phenotype characteristic of low cell density was observed.
Competence
Competence, which is the ability of a microbe to take up DNA from the external environment, is a process that occurs in V. cholerae . The DNA absorbed can then undergo transformation into the genome through recombination. Being naturally competent allows the possibility of horizontal gene transfer (HGT). The presence of large genomic islands in the genome of V. cholerae indicates that HGT has occurred throughout its evolutionary history (8). Transformation could allow for the acquisition of virulence genes by nonpathogenic strains of V. cholerae .
Chitin, found in the exoskeletons on which V. cholerae live symbiotically, is required for the induction of competence and subsequent transformation in V. cholerae (12). The detection of chitin and subsequent alteration of gene expression to allow for competence and transformation is regulated by the protein TFox. However, other factors, such as high concentrations of cyclic-AMP in the cell, are required for transformation to occur. Quorum sensing also has an effect on whether DNA uptake will occur. Both of the autoinducers that V. cholerae produces contribute to the expression of comEA. However, CAI-1 is more important for this process, with AI-2 playing a less significant role (8). The quorum sensing transcription factor HapR is also required for transformation (12). While quorum sensing does not regulate all of the genes involved in transformation, it does regulate two: comEA and comEC. ComEA encodes a DNA-binding protein located in the perisplasm that activates competence-involved genes. ComEC is directly involved in taking up DNA from the external environment.
Quorum sensing also acts to regulate the nuclease dns (12). Under conditions of low cell density, low concentrations of HapR are present and dns is transcribed and translated to protein. This protein is excreted from the cell and acts to degrade free-floating DNA in the environment. Thus, there is not DNA available to recombine into the genome of V. cholerae . The reverse process occurs at high cell density when high concentrations of HapR are present. Less of the nuclease is produced, and as a result, there is a possibility for surrounding DNA to be taken up.
The induction of competence does not happen equally to all V. cholerae cells in a population (12). This disparity is postulated to exist due to unequal distribution of autoinducers in the environment, as well as unequal distribution of the chitin derivatives required to induce competence genes.
Quorum Sensing Cheaters
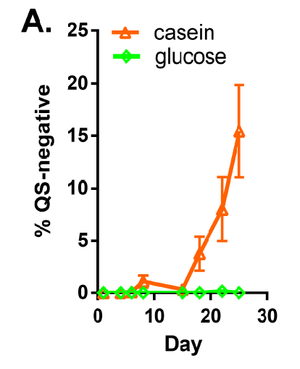
One emerging area of study in quorum sensing is the significance of the role of signaling-deficient bacteria. Quorum sensing depends on individual bacteria expending energy to produce signals and proteins that other nearby individuals are also producing. Thus, it is a pathway that is susceptible to the emergence of social cheaters who capitalize on the shared resources but do not produce any themselves. Genetic analysis of V. cholerae reveals that deficiencies in quorum sensing are coming in V. cholerae ; however, it is less common among pandemic strains (13). In fact, the first genome of V. cholerae to be sequenced, from the strain N16961, was shown to be deficient in quorum sensing (12). The majority of these deficiencies stem from mutations in the genes encoding hapR (10). The evolutionary viability of QS cheaters is reinforced by the fact that clinical and environmental isolates show a variety of mutations in hapR, suggesting that these mutations often occur de novo and are not just transferred between populations. Despite this evolutionary benefit to hapR mutations, there is also a downside in that deficiencies in this gene are associated with reduced competence of V. cholerae . Thus, a fitness trade-off is likely to exist in the acquisition of hapR mutations because these mutations could result in lower ability to take up genetic material from the external environment.
Populations of V. cholerae with a high prevalence of QS mutants produce similar levels of signaling molecules to populations without these mutants, implying that the QS-mutants are signaling other individuals to produce these molecules (10). A relatively low prevalence of organisms capable of producing quorum sensing molecules appears to be sufficient to sustain the biofilm. However, it is thought that the presence of these QS-deficient bacteria could lower the antibiotic resistance of the biofilm and decrease its overall productivity.
Specific environmental conditions are conducive to the emergence of QS mutants, while other sets of conditions do not appear to cause these developments (10). Under conditions of LB, simulated seawater, and pondwater, prevalence of hapR mutations did not increase. However, being a QS mutant is advantageous to V. cholerae when in the presence of wild type organisms in a biofilm. The nutrient availability also plays a role. When V. cholerae are cultured with glucose as a nutrient source, the prevalence of QS mutations does not increase. However, when casein protein is the only nutrient source, QS-mutant organisms do increase in proportion to the entire population, as demonstrated in Figure 3. Interestingly, some mutations observed were identical to those previously sequenced in clinical and environmental samples. Under conditions in which hapR mutants become more common, the total number of cells did not significantly change, indicating that these mutations were not causing large problems for the biofilm as a whole. In addition, quorum sensing mutations were favored specifically under biofilm conditions and not during planktonic growth. This observation is clinically relevant considering that V. cholerae infecting humans is likely to be growing on surfaces and not free-floating.
One mechanism that could explain the advantage of QS deficiency for the individual microbe is that is lowers the expression of extracellular proteases (10). In V. cholerae , HapR activates two proteases, HapA and PrtV, in the process of quorum sensing. A strain with both hapR and PrtV deleted was unable to grown in casein-rich medium. When only one of the proteases was deleted, growth did occur. However, no QS-mutants developed over extended growth of the single protease-knockout strains. This result suggests that the metabolic cost avoided by lacking one of the proteases counterbalances the potential energetic benefit of incurring a QS mutation.
Additionally, there was no difference in the amount of the QS autoinducers AI1 and CAI-1 between colonies with a high presence of QS mutations and those without (10). This lack of difference can be explained by the fact that HapR, the quorum sensing master regulator, does not have an effect on autoinducer levels and is not required for their production. Thus, organisms deficient in HapR would be producing equivalent levels of autoinducers to the wild type.
Social cheating of V. cholerae could be especially relevant in the context of algae blooms (10). Under these conditions, there are high concentrations of dissolved organic matter in the form of proteins and carbohydrates. In addition, the protein found in the fecal matter of V. cholerae hosts could be conducive to the emergence of social cheaters.
Therapeutic Opportunities
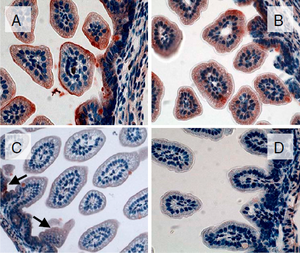
The possibility of antibiotic resistance is a huge concern in the development of new anti-microbial agents. One way to minimize the rise of resistant microbes is to design drugs that alter the behavior of bacteria instead of disrupting cellular functioning to kill the cell (14). The idea behind this approach is that it results in less selective pressure, hypothetically lowering the potential for microbes to evolve to be resistant. Quorum sensing is a process that could theoretically be disrupted in this manner, altering the pathogenesis of a bacterium but not necessarily killing it.
When V. cholerae initially infects a host, there are very low levels of autoinducers present, so the genes for both biofilm and virulence are activated (14). As the bacterial density in the host rises, V. cholerae transitions out of the biofilm phase and becomes less virulent. Proteases that are produced in response to the autoinducer CAI1 cause the bacterium to detach from the walls of the intestine, so it can pass from the host’s system. One possibility to fight cholera infections, then, is to introduce CAI1 into the host which could trigger the deactivation of biofilm and virulence phenotypes.
One approach to introduce autoinducers into the host is through genetically engineered commensal bacteria (15). Nissle, which is a model commensal strain of E. coli , has been transformed to express cqSA, the gene encoding the enzyme that catalyzes the synthesis of CAI-1. It was linked to the promoter fli-C, which is constitutively expressed. Mice that were fed these transformed bacteria and subsequently infected with V. cholerae showed much higher survival rates in a dose-dependent manner. The researchers quantified the number of colony-forming units (CFUs)of V. cholerae present in the intestines of mice that were infected with the cqSA-producing bacteria and those that were not, and found that V. cholerae were less likely to be present in the former group. The administration of the commensal bacteria lowered the accumulation of cholera toxin (CT) as well as lowered the extent of V. cholerae binding to the intestine. Figure 4 shows the lower levels of cholera toxin that accumulate in mouse intestines when the mice are co-infected with E coli expressing V. cholerae cqSA. The duration of the treatment was extremely important for the potential of this system as a prophylactic, as pre-treatment at 8 hours was much more effective than at 4 hours. A similar prophylactic approach could be used as a preventive measure where the possibility of cholera epidemic is high, such as areas that have recently experienced disruption of infrastructure due to a natural disaster. A limitation to this approach is that adult mice are not susceptible to lasting infection by V. cholerae , so the window of study is short.
An alternative to infection with live transformed bacteria would be an oral nanoparticle therapy where CAI-1 is covered in a soluble coating to improve its delivery. One difficulty in designing an oral therapy for CAI-1 is that it is a large, hydrophobic molecule (14). As a result, it would be challenging to deliver it at a high enough concentration to have a therapeutic effect. To address this problem, nanocarriers have been considered as an alternative. This approach involves coating the hydrophobic molecule with polyethylene glycol (PEG). Nanoparticles improve the kinetics of the drug, allowing it to be better solubilized. It also aids its passage through mucus layers. Flash Nano Precipitation was used to make the CAI-1 nanoparticles, a protocol which has been employed in other medicinal studies to improve the kinetics of hydrophobic drugs. In order to effectively target V. cholerae , a nanoparticle would have to be impermeable to acid so it could pass through the stomach to the small intestine without being degraded. The bile micelles in the small intestine then dissolve the CAI1 particles, releasing the contents, while also facilitating transport across the mucus layer.
In order to test the effectiveness of this potential therapy, the creators of the nanoparticle system used a reporter strain of V. cholerae that cannot produce its own CAI-1, but does have a luciferase insert that is activated in the presence of external CAI-1. This pathway, which results in bioluminescence, is the same cascade for biofilm and virulence gene expression. However, the effect is reversed, because bioluminescence is activated by CAI-1 while the biofilm and virulence phenotypes are repressed. These assays demonstrated that there were higher levels of bioluminescence produced in the presence of nanoparticle CAI-1 than free CAI-1. Thus, nanoparticles are a promising avenue for delivery of autoinducers as a therapeutic.
References
[2] CDC - Topic Title - Healthy Water. (2015).
Authored for BIOL 238 Microbiology, taught by Joan Slonczewski, 2015, Kenyon College.