The Skin Microbiome and Malaria
Introduction
By Aldis Petriceks
Double brackets: [[
Closed double brackets: ]]
Bold
Italic
Subscript: H2O
Superscript: Fe3+
Malaria is a deadly disease caused by the protozoan Plasmodium, and spread through mosquito bites. [1] The most common ailments are fever, chills, and flu-like symptoms which typically begin within a month of infection [2]. Other symptoms include headache, vomiting, jaundice, shivering, and joint pain. The most notable sign of a malaria infection is paroxysm – which includes cycles of cold and shivering followed by fever and sweating. While immediate treatment can typically facilitate a full recovery, those without access to medical care can fall fatal to the disease within days. There are currently 3.4 billion people living in high-risk areas, which led to 500,000 deaths from malaria in 2013 alone. The majority of these deaths occur in underdeveloped tropical and sub-tropical countries, which in turn incur the bulk of the $12 billion USD yearly cost associated with fighting the disease.
Malaria progresses in two stages. In the first stage, the Plasmodium parasite (in sporozoite form) is injected into the host bloodstream by a mosquito vector, where sporozoites then travel to the liver [3]. Once inside liver cells, these sporozoites spend the next 5-16 days dividing at rapid rates into haploid merozoites. Merozoites build-up in the liver cells causes cell rupture, which then releases the merozoites back into the bloodstream. This begins the second stage of the disease, where the parasites infect red blood cells, undergo many cycles of asexual reproduction, and cause their new host cells to rupture as well, further propagating merozoite production. Infection and rupture cycles repeat every 1-3 days, which manifest themselves in the shivering and fever cycles mentioned above [4].
While the most commonly-known risk factors for malaria are geographic location, socioeconomic level, and lack of proper medical care, research suggests that an individual’s skin microbiome can heavily influence attractiveness to malaria-carrying mosquitoes [5]. Odorous chemical cues are key to mosquito orientation and landing during host location, and skin flora play a large role in the process by affecting human odor production. Specifically, these microbes metabolize lipids and other compounds in naturally odorless sweat, producing volatile odor compounds attractive to anthropophilic mosquitoes [6]. Skin bacteria vary widely in their metabolic pathways, and thus have differing volatile odor productions. As such, composition of skin flora can have large effects on host location in such mosquitoes.
The Skin As An Ecosystem


The skin is the largest organ in the body, and constitutes over 1.8 square meters of surface area in which microbes can form habitats. [7] Bacteria, fungi, viruses, and microbial eukaryotes are all common inhabitants of this interface with the outside environment. Similar to any ecosystem, the skin microbiome contains symbiotic organisms of many different natures – many are harmless, others are beneficial to human health, and some are pathogenic and facilitate disease. [8]
The overall environment of the human skin is typically cool in temperature and slightly acidic [9]. However, taking into account the many regions of folds, invaginations, and hair, specific habitats can vary widely between regions of the body. This is especially true in and around the sweat glands. For example, sebaceous glands promote growth of facultative anaerobes such as Propionibacterium acnes due to their oxygen-poor environment. [10]
Relative humidity, temperature, and light also varies widely among body regions. Unsurprisingly, these effects can exert great influence on bacterial composition. The skin microbe S. aureus flourishes in the axillary and groin regions, both of which are high humidity and temperature, and low in light exposure. [11] As such, S. aureus is able to grow in high proportions in these environments. On the other hand, most bacterial strains are not able to grow as successfully in dry and open regions such as the arms and legs.
Considering the influence of topography, temperature, humidity, light, sweat gland, and washing, it is difficult to describe a single, overarching microbiome within an individual’s skin. Rather, the skin microbiome can be thought of as several smaller ecosystems, each of which applies its own specific selection pressures. For example, the scalp is high in proportions of sebaceous glands, which strongly selects for facultative anaerobes and lipophiles such as Propionibacterium. [12] This strong selection pressure results in a relatively low species diversity around the sebaceous glands. In moist regions such as the gluteal crease or sole of the foot, Staphylococcus and Corynebacterium tend to dominate, as they are adept at metabolizing the lipids and proteins present in apocrine sweat.
While dry, desiccated skin such as that of the arms or legs tends to support less-abundant microbial life than warm, moist regions, these areas provide the greatest levels of bacterial diversity throughout the human skin microbiome. One hypothesis for this phenomenon is that the lack of heat and humidity prevents any single bacterial species from flourishing and thus dominating other species, allowing for smaller proportions of many different bacteria to remain. [13]
Anthropophilic Mosquito Olfaction

Mosquitoes rely heavily on olfaction during orientation and landing. Olfactory receptors are based in the antennae and maxillary palpi, with each site containing receptors for specific odor compounds [14]. Upon contact with such compounds, olfactory cells send electrical signals to higher-processing centers in the olfactory system [15]. In anthropophilic mosquitoes, these cues typically come in the form of sweat metabolites, which are given their odor by bacteria on the skin epithelium. Studies suggest anthropophilic mosquitoes have evolved to recognize sweat metabolites from human skin bacteria, as these insects greatly prefer natural human scents to synthetic blends of similar salt, water, and heat composition [16]. As variation exists among individuals’ skin flora compositions, relative attractiveness to mosquitoes also differs between humans.
Short-chain carboxylic acids, thioalcohols, benzaldehyde, and other compounds are known to be particularly attractive to anthropophilic mosquitoes [17]. This is because such compounds are common byproducts of volatile-producing skin bacteria, and thus signify nearby presence of a human host. Studies suggest that compounds which are unique to human scent are more attractive to these mosquitoes than more commonly found odor compounds [18].
Specific genes have been found to code for olfaction of human sweat odorants in anthropophilic mosquitoes. For example, the AgOr1 gene codes for mosquito sensory identification of the odorant 4-methylphenol. AgOr2 codes for olfaction of 2-methylphenol, a less-common metabolite of human skin flora It is worth noting that such genes are expressed almost exclusively in female mosquitoes, and transcription of these genes decreases significantly after a blood meal [19].
Regardless of specific olfactory genes, mosquitos can typically detect human odor molecules at short ranges for several weeks after production [20]. Furthermore, anthropophilic species such as the malarial vector Anophiles gambiae Giles sensu stricto prefer human odor compounds over those of other vertebrate species. On the other hand, species such as An. quadriannulatus are preferential towards the scent of cows and other non-human animals.
Microbial Production of Body Odor
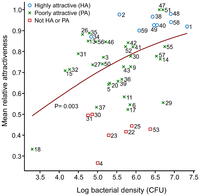
Aerobic coryneforms such as Micrococcaceae and Propionibacterium are the most abundant volatile-producers in high-odor regions such as the underarm [21]. Odor intensity typically increases with coryneform abundance and density, more so than in other skin epithelium bacteria such as Staphylococcus. However, there is still debate as to which bacteria exhibit the strongest association with mosquito attraction.
Attractive bacteria produce the well-known sweat odor by metabolizing lipids and other compounds present in human sweat [22]. Byproducts of this metabolism such as propionic acid, thioalcohols, and benzaldehyde are highly aromatic and give sweat its characteristic, unpleasant smell. Propionic acid, for example, is a product of amino acid breakdown by Propionibacterium. Isovaleric acid is a common metabolic byproduct of the commensal Staphylococcus epidermidis [23].
Because specific volatile production is dependent on the specific metabolic pathways of skin epithelial bacteria, different skin flora compositions can lead to varying odor emanations from the human body. Odor production of individual bacterial strains produce depend largely on the strain’s abundance and capacity to metabolize fatty acids in human sweat. Aerobic coryneforms typically have a high correlation with volatile odor production in humans, owing to their high abundance near sweat glands, and ability to metabolize lipids into free carboxylic acids [24].
Volatile-producing bacteria contain specific genes associated with production of thioalcohols, short-chain carboxylic acids and other volatile compounds. These genes often code for enzymes such as lipases, which metabolize lipids and fatty acids by breaking them down into smaller, more volatile compounds [25]. Interestingly, volatile compound production may vary between growth phases in an individual bacterial species. For example, volatiles produced by skin epithelial bacteria in stationary phase were found to be more than those produced in log phase growth. While a partial explanation for this pertains to the increased bacterial population during stationary phase, analysis also suggests different volatile compounds are produced during different growth phases [26]
Although the large majority of volatiles produced by skin bacteria originate from the metabolism of lipids, breakdown of aliphatic amino acids can also produce malodorous compounds [27]. Volatile compounds produced through this metabolic pathway are typically the same or similar to compounds produced by lipid catabolism. These include short-chain carboxylic acids such as 3-methylbutanoic acid or propanoic acid.
Anthropophilic Mosquito-Attracting Bacteria

Considering that a bacterium’s specific pathways for lipid catabolism is key to the specific odor produced from sweat, it is no surprise that mosquito location is dependent on the presence of specific volatile-producing bacteria. Individuals with higher proportions of these attractive bacteria can lead to increased mosquito location and biting.[28] This attractiveness has little to do with the bacteria themselves, but rather the compounds they produce. These compounds are sufficient for attraction, as mosquitoes will preferentially land on agar plates coated with them. In addition, research suggests that the specific odors which attract such mosquitoes are almost exclusively of bacterial origin [29]. This is evidenced by the fact that washing certain body parts with bactericidal soap significantly decreases mosquito host location in those regions.
Certain compounds may be attractive to mosquitos because they are produced by bacteria which are specific to vertebrate skin flora. [30] For example, Corynebacterium minutissimum is a common colonizer of human skin, yet is uncommon elsewhere. The sweat metabolites produced by this bacterium are thus highly attractive to anthropophilic mosquito species.
Anophiles gambiae Giles sensu stricto, one of the main vectors for Malaria in sub-Saharan Africa, has shown differential attraction to several different volatile compounds produced by skin flora.[31] When exposed to individual human scents (in used socks), An. gambiae will prioritize scents derived from humans with high proportions of Acidobacteria, Delftia, and Leptotrichia. In addition, increased overall bacterial abundance correlated with increased attractiveness to An. gambiae. On the other hand, bacterial diversity is negatively correlated with An. gambiae attraction.
While volatiles produced by Staphylococcus, Acidobacteria, Delftia, Leptotrichia, and Propionibacteria are highly attractive to anthropophilic mosquito species, those produced by Pseudomonas and Variovorax bacteria are relatively unattractive.[32] Studies suggest that these poorly-attractive bacteria might actually decrease mosquito host location through a number of mechanisms. These include metabolism of volatile compounds produced by attractive bacteria, inhibitive signaling to volatile-producing bacteria, production of compounds which actively repel mosquitoes, and masking of volatile compounds present on the human skin [33]. This may explain why increased bacterial diversity is negatively correlated with An. gambiae attraction: Increased heterogeneity allows for greater proportions of poorly attractive bacteria, which counteract volatile production of attractive bacteria and inhibit mosquito host location. An analogous example is the role of gut bacteria in the human immune system. As such, these beneficial skin bacteria can be thought of as a “built-in defense system” against anthropophilic mosquito location.
Identification and Characteristics of Mosquito-Attracting Bacteria
Aerobic coryneforms (one of the most attractive groups of bacteria to anthropophilic mosquitoes) are Gram-positive, rod-shaped microbes. This taxa includes many bacterial genera, including Bacillus, Lactobacillus, Kurthia, Listeria, and Erisypelothrix.[34] While coryneforms are wide-ranging in there metabolism, those that produce volatile odors on human skin are typically classified as lipophilic coryneforms. These bacteria live in and around sweat glands in order to remain close to these sources of lipids and fatty acids. Dermabacter and Propionibacterium are two prominent lipophilic skin coryneforms, and both associated with high attractiveness to anthropophilic mosquitoes.[35]
Staphylococci, another major group of mosquito-attracting bacteria, are spherical, Gram-positive, and organize their cells into clusters. This genus is composed of several well-known skin microbes, such as S. epidermidis, S. aureus, and S. haemolyticus MicrobeWiki. "Staphylococcus."</ref>. While odorous compounds produced by Staphylococci are not typically as volatile as those of coryneforms, large numbers of these bacteria on human skin tend to increase mosquito sensation of produced odors. [36]
Factors Affecting the Skin Microbiome
Many factors, both genetic and environmental, combine to influence the volatiles produced by sweat-metabolizing skin microbes. In particular, genetic influences are especially important for skin flora composition. For example, dogs often have significant difficulty in distinguishing between the odors of identical twins, even those raised in separate families.[37] It is also likely for genetic reasons that human individuals tend to show preference for human odors more different from themselves.
One way in which genetics can manipulate human odor emanation is by affecting the composition of sweat itself. Those who produce fewer odor precursors in their sweat are less likely to attract mosquitoes, because their skin flora cannot produce volatile compounds in as great numbers. For example, less than two percent of the human population secretes apocrine and sebaceous sweat which completely lacks odor-precursor lipids and amino acids, and thus their skin bacteria do not metabolize such compounds into volatile forms. Absence of the ABCC11 appears to be the cause of this unique phenotype. [38] Specifically, homozygous genotypes in the SNP (538G>A) tend to result in such odorless sweat, at trait which most commonly manifests itself in Asian populations.
Apart from genetic factors, environmental influences can also serve to manipulate microbial production of volatile compounds. One intuitive cause of increased volatile production is increased exercise, which increases perspiration. While most exercise-induced sweating is done through the eccrine glands, augmented exertion is also associated with greater secretion of lipids and oils from apocrine and sebaceous glands [39]. As such, by providing an increased source of lipids and fatty acids, exercise can induce greater levels of volatile compounds on human skin.
Food consumption may also affect human odor production, and thus attraction to mosquitoes. These effects are typically caused by the presence of certain odorous compounds present in specific foods. For example, foods which are high in sulfur (such as eggs, broccoli, and cabbage) may cause sulfur-containing compounds to be released in the sweat, causing odor even prior to microbial action [40]. However, because most mosquito species locate human hosts via olfaction of aliphatic carboxylic acids, production of these sulfurous odors may not increase attractiveness to such anthropophilic species.
Use of body soap and deodorant have both be analyzed deeply for their effects on the human skin flora. However, to this point, the effects of such products on skin bacteria are unknown, beyond the fact that they alter skin flora composition. [41]
References
- ↑ CDC, (2016). "Malaria"
- ↑ Caraballo, H (2014). "Malaria, Dengue, and West Nile Virus"
- ↑ NIAID (2016). "Malaria"
- ↑ NIAID (2016). "Malaria"
- ↑ Carde, H & Gibson, G (2016). "Host finding by female mosquitoes: mechanisms of orientation to host odours and other cues."
- ↑ Olanga et al (2016). "Attraction of Anopheles gambiae to odour baits augmented with heat and moisture."
- ↑ Grice, E & Segre, J (2016). "The skin microbiome."
- ↑ Grice, E & Segre, J (2016). "The skin microbiome."
- ↑ Grice, E & Segre, J (2011). "The skin microbiome."
- ↑ Grice, E & Segre, J (2011). "The skin microbiome."
- ↑ Rennie et al (1991). "In-vitro and in-vivo studies of human axillary odour and the cutaneous microflora."
- ↑ Grice, E & Segre, J (2011). "The skin microbiome."
- ↑ Rennie et al (1991). "In-vitro and in-vivo studies of human axillary odour and the cutaneous microflora."
- ↑ Takken, W & Knols, B (1999). "Odor-mediated behavior of Afrotropical malaria mosquitoes."
- ↑ Zwiebel, L & Takken, W (1999). "Olfactory regulation of mosquito-host interactions."
- ↑ Olanga et al (2010). "Attraction of Anopheles gambiae to odour baits augmented with heat and moisture."
- ↑ Olanga et al (2010). "Attraction of Anopheles gambiae to odour baits augmented with heat and moisture."
- ↑ Hallem et al (2004). "Olfaction: mosquito receptor for human-sweat odorant."
- ↑ Hallem et al (2004). "Olfaction: mosquito receptor for human-sweat odorant."
- ↑ Zwiebel & Takken (2004). "Olfactory regulation of mosquito-host interactions."
- ↑ Rennie et al (1991). "In-vitro and in-vivo studies of human axillary odour and the cutaneous microflora."
- ↑ Lundstrom & Olsson (2010). "Functional neuronal processing of human body odors."
- ↑ Lundstrom & Olsson (2010). "Functional neuronal processing of human body odors."
- ↑ Taylor et al (2003). "Characterization of the microflora of the human axilla."
- ↑ Verhulst et al (2011). "Composition of Human Skin Microbiota Affects Attractiveness to Malaria Mosquitoes."
- ↑ Verhulstt et al (2011). "Composition of Human Skin Microbiota Affects Attractiveness to Malaria Mosquitoes."
- ↑ Smallegange et al (2011). "Sweaty skin: an invitation to bite?"
- ↑ Verhulst et al (2011). "Composition of Human Skin Microbiota Affects Attractiveness to Malaria Mosquitoes."
- ↑ Verhulst et al (2011). "Composition of Human Skin Microbiota Affects Attractiveness to Malaria Mosquitoes."
- ↑ Verhulst et al (2010). "Differential Attraction of Malaria Mosquitoes to Volatile Blends Produced by Human Skin Bacteria"
- ↑ Verhulst et al (2010). "Differential Attraction of Malaria Mosquitoes to Volatile Blends Produced by Human Skin Bacteria"
- ↑ Verhulst et al (2010). "Differential Attraction of Malaria Mosquitoes to Volatile Blends Produced by Human Skin Bacteria"
- ↑ Verhulst et al (2011). "Composition of Human Skin Microbiota Affects Attractiveness to Malaria Mosquitoes."
- ↑ Funke et al (1997). "Clinical microbiology of coryneform bacteria."
- ↑ Funke et al (1997). "Clinical microbiology of coryneform bacteria."
- ↑ Funke et al (1997). "Clinical microbiology of coryneform bacteria."
- ↑ Kuhn (2009). "Body odour of monozygotic human twins: a common pattern of odorant carboxylic acids released by a bacterial aminoacylase from axilla secretions contributing to an inherited body odour type"
- ↑ Preti & Leyden (2010). "Genetic influences on human body odor: from genes to the axillae."
- ↑ American Skin Association. "Healthy Skin."
- ↑ WebMD. "6 Tips for Reducing Body Odor."
- ↑ Grice, E & Segre, J (2011). "The skin microbiome."
Authored for BIOL 238 Microbiology, taught by Joan Slonczewski, 2016, Kenyon College.