Tobacco Mosaic Virus Uses In Pharmaceutical Research
Tobacco Mosaic Virus Uses in Pharmaceutical Research
By Michael Itschner II
Introduction
The Tobacco Mosaic Virus (TMV) has been well researched since it has been defined a virus in 1898 by Martinus Beijerinck.[1] TMV has been used as a model virus for a teaching tool in biology classes and as a scaffold for drug delivery mechanisms.[6] Since there has been much research done on this virus previously, the structure of TMV has been extensively studied and is well understood.[5] The scaffolding of this virus is of particular interest because its protein base serves as a template for precise targeting of surface groups on cells. Plant viruses have drawn more attention since they are unable to infect mammalian hosts and have good blood and tissue compatibility in mammals.[5,6] TMV is also biodegradable and able to be cleaned out of non-target tissues and organs, making TMV a promising candidate for the targeting and delivery of therapeutics to mammals.[5,6]
Discovery
The discovery of the Tobacco Mosaic Virus (TMV) and the mosaic disease it caused can be attributed to three individuals, Adolf Mayer, Dmitrii Iwanowski, and Martinus Beijerinck. In 1886 Adolf Mayer (1843-1942) found in his research that extracts from ground up diseased tobacco plants could infect healthy tobacco plants. He followed Koch’s postulates moving forward in his research and was able to produce cultured organisms from the extracts but would not be able to reproduce the disease. Using filter paper to screen out cellular pathogens, Mayer concluded that a bacterial species was responsible. [1]He reasoned that the infectious agent passed through the initial filtration paper, but after multiple filtrations the agent stopped infecting other plants. Replicated research on the filtration of the microbe causing the tobacco mosaic disease by Dmitrii Iwanowski (1864-1920) found contrasting results to Mayer[1]. In 1892 Iwanowski reported that the Chamberland filter candles, which were fine filer that retained bacteria, did not trap the disease-causing microbe, it passed through the filter. This led Iwanowski to believe that a toxin released from a bacterium, which was dissolved in sap, caused the disease or the bacterium was small enough to pass through the filter candles [1]. Martinus Beijerinck (1851-1931) also worked with filtration experiments and also found that the material, absent of microorganisms, that passed through the porcelain filters continued to be infectious to healthy plants. In 1898 Beijerinck called this material a virus and continued to research its transmission and growth. Although these three individuals were not completely correct in their assumptions about the TMV, their discoveries were crucial to its classification in the microbial world.[1]
Structure
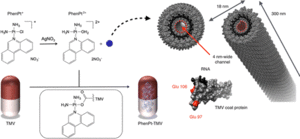
The crystal structure of TMV was discovered in 1935 by Wendell Stanley (1904-1971), which led him to win the 1946 Nobel Prize in Chemistry for the first crystallization of a virus.[2][3]TMV is rod-shaped and is arranged in a helix of repeating protein subunits with its single stranded RNA embedded within it, resembling a nanotube.[4]One TMV particle has 2,130 copies of the protein that forms the coat of the virus, which covers the 6,400 single stranded RNA nucleotides.[5] The TMV RNA contains the genetic information for four genes, two of which are replicase-associated proteins, a movement protein and a coat protein.[5] The RNA is coiled in a positive sense orientation acting as an mRNA in the host using the host ribosomes to translate its proteins and continue its reproduction.(Figure 1)
Transmission
It is easy for TMV to spread between tobacco plants, and other related plants, through direct contact such as leaf to leaf, or through indirect contact such as tools touching an infected plant and then touching a healthy one. One damaged tobacco plant cell is all it takes for a TMV particle to enter the organism and begin replication. Tobacco seed coats can also be contaminated with this virus, infecting the germination of the plant.[5] TMV is also very stable and can still infect plants causing the mosaic disease for many years after its release. A study found that a 50 year old TMV that had been in storage at 4 °C could still infect tobacco plants.[5] Once the TMV has used the host ribosomes to synthesize the replicase-associated proteins, they attach themselves to the 3’ end of the positive sense strand of the RNA and begin the synthesis of the negative sense strand of RNA.[5] The negative sense RNA acts as the template strand for the synthesis of a full positive sense genome and subgenomic RNA (sgRNA). TMV uses its sgRNA and the host translational machinery to translate its movement protein (MP) and coat protein (CP), weighing approximately 30 kDa and 17.5 kDa respectively. When the coat proteins are synthesized they associate with the new positive sense RNA producing viable virions that can infect other healthy tobacco cells within the plant.[5] Movement proteins are used to transport the viable TMV virons to neighboring cells through the plasmodesmata between the plant cell walls. Under normal conditions the plasmodesmata is too small for the whole TMV complex to pass through, but when bound to the movement proteins the plasmodesmata expands its diameter and allows the TMV virion to pass through the junction and infect the neighboring cell.[5] This process is repeated for cell-to-cell infection, but once the virus particles reach the plants phloem, the vascular tissue in plants, the infection becomes systemic, spreading from the roots to the leaves.[5]
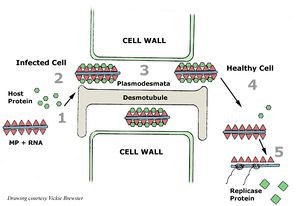
Epidemiology
Viruses need to have a host organism in order to continue having progeny and passing on their genetic code, TMV is no different. The host plants can be infected by TMV entering the plant through a damaged cell or contamination of virions on healthy seed coats, possibly from farmers not being careful when handling TMV contaminations. The virus can spread via clothing and tools on farms and in greenhouses.[5] Since TMV particles are stable, they can survive in dead plant material over many months and through harsh weather conditions. This virus can also be a recurring problem if farmers plant the same crops year to year.[1](Figure 2)
Using TMV in Drug Delivery
Investigations looking into viruses as suitable carriers for cancer therapeutics have been gaining in popularity in recent years. Viruses have evolved to effectively spread their genes into host organisms for millions of years, making them logical candidates to use as delivery vectors of anticancer drugs to specific tissues. TMV has been increasing in popularity as delivery tool because plant based viruses are unable to infect or replicate in mammalian cells, and TMV is biocompatible in human blood and tissue.[6] TMV is also biodegradable, clearing from non-target organs in a few hours[6]. In the field of nanocarriers, aspect-ratio (AR), the length of a molecule divided by its width, is a statistic that is used to determine the efficiency of a nanocarrier to transport its drug cargo its destination. The dimensions of TMV used in the nanocarrier studies are 18 nm wide and 300 nm long with a 4 nm wide channel in the middle of the tubule [6]. A high AR indicates that the carrier will have favorable targeting interactions and better distribution of its payload. The nanotube structure of TMV offers a high AR, which is good for evading the immune system, and has more efficient targeting interactions with tumor cells[6]. Many people who receive chemotherapy are given drugs that are platinum based agents. Toxicity and drug resistance are major drawbacks for this category of drug[6]. Off target toxicity from therapeutics results in the damage of healthy tissue, causing patients to fall ill to preventable diseases, fatigue, nausea and many more side effects[7]. Cancer cells that do not fully terminate can persist on living causing recurring cancers and mutate into drug resistant forms[6]. Using a carrier to hold the therapeutic drugs can reduce the toxic side effects of the drug. Carriers can be used as a targeting apparatus to bring the drugs to the cancerous tissue without any degradation of the drug to biological processes. Phenanthriplatin is a drug that is up to 40 fold more potent that other platinum agents, but its unable to be more effective than this class of drug because there is no efficient delivery mechanism established[7].
TMV as a Treatment for Melanoma
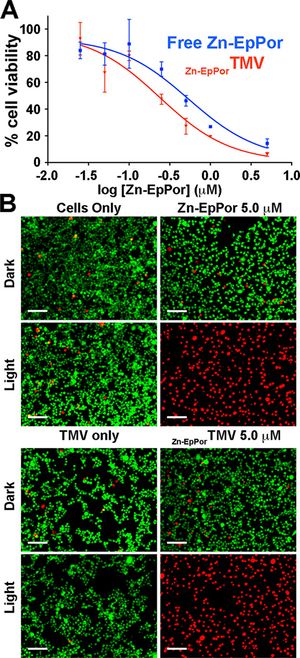
Photodynamic therapy (PDT) is a different approach to treating melanoma, cancer of the skin. There are three requirements of this method and they are all nontoxic, a photosensitizer, light, and oxygen[8]. The combination of these three requirements in a cell will lead to cell death. When the photosensitizer is exposed to a certain wavelength of light in the presence of oxygen it will begin to react, resulting in the release of a reactive oxygen species (ROS)[8]. The ROS will cause damage to the internal cellular components by oxidative stress causing the cell to undergo apoptosis. Photosensitizers are not soluble under biological conditions and do not accumulate in high enough concentrations in tumor tissues to be effective, and patients have to avoid sunlight after treatment to prevent unwanted activation the drug. The use of TMV as a delivery system for the photosensitizers offers a promising method to increase concentrations in tumor tissues and lower off target side effects[8].
The interior of the TMV has a high concentration of negative charges due to the glutamic acids that are exposed in this area. Research done by Lee et al. (2016) has capitalized on this detail using a photosensitizer, Zn-EpPor, which has positive charge molecules to load into the TMV based on charge-charge interactions[8]. The positive Zn-EpPor will bind favorably to the negatively charged internal TMV tube. They found that approximately 800 molecules of their photosensitizer were successfully loaded into the TMV structure. Using fluorescence analysis, they found that there was a 40% increase in cellular uptake of the Zn-EpPor TMV than the Zn-EpPor alone in B16F10 melanoma cell lines[8]. They report that when the melanoma cells take up the Zn-EpPor TMV, they are transported to the endolysosomes[8]. The acidic environment in these organelles case the protonation of the carboxylic acids in the interior of the TMV and giving it a more positive charge and triggering the release of the Zn-EpPor drug[8]. When this drug is then exposed to light, it begins to release ROS molecules causing the cell to die. After the release of the drug, the TMV shell will then be degraded by the proteases and hydrolyases that are contained within the lysozymes[8]. The degraded parts are then excreted with the other waste products from cellular function. TMV works well as a nanocarrier in this field because of its effective delivery to target cells and release its payload while being able to be degraded and excreted without having any harmful side effects.(Figure 3)
Tobacco Mosaic Virus (TMV) as a Vaccine
Francisella tularensis is a pathogenic bacterium that is responsible for the disease tularemia, also known as rabbit fever. The Center for Disease Control (CDC) has documented this pathogen as a biological threat. Several countries have studied this bacterium as a bioweapon for biological terrorism[9]. The grounds for F. tularensis to be considered a biological weapon due to the fact that it only takes a few cells to cause infection, can be released as a vapor, incapacitates its hosts, and causes death in a short period of time[9]. Previous attempts to develop a vaccine have not been successful, with the closest usable vaccine being a Live Vaccine Strain (LVS) that was made using F. holartica from Russia[9]. While this vaccine did offer some resistance to tularemia, it still caused health problems and negative reactions in its patients, forcing the US Food and Drug Administration (FDA) to turn down approval[9]. Currently, a vaccine has not been developed yet for the prevention of this disease. Research by Banik et al. (2015) has investigated the potential of using the tobacco mosaic virus as a delivery apparatus to transport a potential vaccine molecule to combat tularemia.
The failure of these previous vaccines can be attributed to their design being a single subunit of a larger structure was aimed at targeting the bacteria, but was not able to defend against all the possible variations of infectious F. tularensis strains[9]. Another possible explanation for the vaccine failures could be that single targeting proteins are unable to be affective against all the possible orientations of the bacterial proteins, or a lack of the proper antigens to stimulate an immune response that would target the bacteria[9]. A new approach to developing a vaccine against this disease is two fold; the first being to develop a vaccine that is effective against F. tularensis, and second is to use TMV as its delivery mechanism[9].
TMV is of particular interest as an antigen carrier because its structure allows for it to be ingested by cells easily and is able to display antigens on its surface[9]. Since TMV is able to simulate antibody production could be due to the exposed repeated antigens on its surface. This mimics other viral coats making the human body interpret it as threatening. Or the other possibility is that the TMV RNA stimulates the cell-mediated immunity cascade [9]. Another positive of using TMV as a vector for the vaccine drugs is the fact that TMV cannot infect mammals nor does it have negative affects on host antibodies. TMV can be used several times to boost the vaccine effects also.
To test this combination of TMV with a vaccine drug, Banik et al. (2015) used recombined proteins from F. tularensis in order to stimulate an immune response in mice. They used two different combinations, three different recombinant proteins from F. tularensis inside a TMV (multiconjugate), and a TMV with one type of recombinant F. tularensis protein (monofonjugate)[9](Figure 4). They found in their research that when TMV was paired with these recombinant proteins, an immune response was activated. Antibodies formed against the three recombinant proteins that were used in the experiment. The TMV-monoconjugate had less antibodies being produced than the amount of antibodies produced in the TMV-multiconjugate response[9]. The implications of these results could lead to the formation of a vaccine against bacteria that can be used to inoculate an entire population. This result would also provide humanity with a method to combat an outbreak of a deadly biological weapon in the event of military hostilities escalating to chemical warfare.
TMV Scaffolds Used to Make Other Nanoparticles
Nanoparticles are well sought into because of their applications in drug delivery and in other nanotechnologies. The tobacco mosaic virus is a symmetrical rod of repeating subunits of protein stacked on top of each other, which has the ability to be reformed into other nanoparticle shapes. Research into diverse nanoparticle shapes and forms are being looked into to see their potential use in in vivo studies[10]. Research has found that when TMV rods are heated up they can take on different structures and sizes of spherical nanoparticles. Theses different sized nanoparticles have the potential to be used in the medical field to find a variety of delivery and imagining agents[10]. The spherical nanoparticles form as a result of heating the TMV to 94 °C for at least ten seconds. The size of the nanoparticles can vary depending on the concentration of the TMV that are in solution at one time[10]. The reported sizes of these spherical nanoparticles are between 50-800 nm in diameter and can range in morphology by changing the temperature and time under heat conditions[10]. For the complete conversion of TMV into the spherical nanoparticles, 125 seconds at 100 °C is required. Shorter times at 100 °C will result in a mix of TMV rods and the spheres, and longer times at 100 °C will not change the morphology of the spherical nanoparticles. This indicates a transition threshold at 100 °C[10]. Further investigation into these different nanoparticle shapes could lead to novel structures that could be used for medical applications in drug delivery that researchers are not yet aware of.
TMV Applications in Micromachines
Small-scale machines and batteries, micromachines and microbatteries, have modern day applications in medical devices, commercial electronics, and wireless sensors that are used in environmental, homeland security, and structural purposes[11] Like all other technology, energy is required to make it function, but with the miniaturization of technology, the source of power for these devises also needs to get smaller. The most desired batteries or energy generators are able to have a large energy output with minimal area taken up within the device[11]. Research that has been investigating the improvement of batteries at the microscale level has focused heavily on microelectromechanical systems (MEMS)[11]. This technology uses electrodes that are three-dimensional, opposed to two-dimensional films, to increase the surface area of the electrodes for more reaction space. Another approach to improving battery function at the nanoscale level is the use of nanomaterials to increase the area of space that the electrode and electrolyte meet, increasing the reaction area[11]. Increasing the stability of these chemicals on the small scale also increases the efficiency of the microbatteries[11]. The tobacco mosaic virus is useful in this technology as a scaffold for the production of nanomaterials.
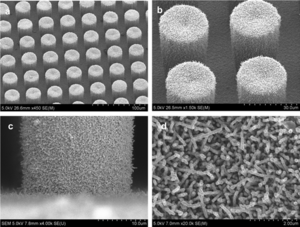
The TMV is more effective in this role when it’s modified with extra cysteine amino acid residues[11]. The thiol functional groups improve TMV’s ability to bind (self assemble) to metal surfaces of the electrodes. When the TMV adheres to the surface of the electrodes they form a strong network that increases the surface area of the electrode for more reactions to take place with the electrolytes[11]. This structure also helps to stabilize the microbattery as a whole. TMV is able to conduct electrons when a metallic film covers the virus to enhance its conductivity. V2O5 was one of these materials used to increase TMV’s conductivity[11]. Underneath the TMV structure, a metal such a gold, nickel or copper, is used to collect the electrons of the reactions from the surface between the conductive TMV and electrolyte solution[11]. In this battery, gold was used as the material of choice to form the electrode pillars. The reasoning for this choice was due to the fact that TMV was able to self assemble onto the gold pillars easily, and it is an inert metal at the voltage range that the battery was operated in (2.6-3.6 V)[11](Figure 5). The implications of these experiments can lead to the development of microscale batteries that have the capability to power small machines; which can be used to for commercial, medical, and military applications.
Conclusions
The tobacco mosaic virus has been studied and documented for well over a century. Its origins of research are based on its infectious pathogenic nature in several species of plants. The structure of TMV, hollow rod of repeating protein subunits surrounding a single RNA strand, allows for researchers to take advantage of nature’s creation and utilize it for other purposes. The hollow nanotube structure of the TMV offers a place for therapeutic drugs to be held and then delivered to its target destination, such as tumors and cancer cells. It’s fascinating that this virus started its relationship with humankind as a killer of crops, but now its being used for many different applications to solve human problems.
References
- ↑ The Discovery of the Causal Agent of the Tobacco Mosaic disease
- ↑ Microbiology, An Evolving Science
- ↑ Tobacco Mosaic Virus
- ↑ The tobacco mosaic virus particle: structure and assembly
- ↑ Tobacco mosaic virus
- ↑ Tobacco Mosaic Virus Delivery of Phenanthriplatin for Cancer therapy
- ↑ Chemotherapy Side Effects
- ↑ High Aspect Ratio Nanotubes Formed by Tobacco Mosaic Virus for Delivery of Photodynamic Agents Targeting Melanoma
- ↑ Development of a Multivalent Subunit Vaccine against Tularemia Using Tobacco Mosaic Virus (TMV) Based Delivery System
- ↑ Nanomanufacturing of Tobacco Mosaic Virus-Based Spherical Biomaterials Using a Continuous Flow Method
- ↑ Hierarchical Three-Dimensional Microbattery Electrodes Combining Bottom-Up Self-Assembly and Top-Down Micromachining
Authored for BIOL 238 Microbiology, taught by Joan Slonczewski, 2018, Kenyon College.