Phage Mediated Biocontrol of Food Borne Bacteria

Introduction
Food borne bacteria exist in all forms of foods humans consume on a daily basis. The control of bacterial pathogens present on fresh fruit and vegetables and ready to eat foods are of major concern since these foods do not generally undergo any further processing or cooking that would kill pathogens before consumption (4). A key reservoir for many human bacterial pathogens is livestock because animals are also subjected to bacterial infections and are contained within relatively enclosed environments (2).
[Phage therapy] is the application of bacteriophages to bacterial infections of humans or animals with the goal of reducing bacterial load (2). Bacteriophages are a bacterial parasite, ubiquitous in environment, and can infect over 140 bacterial species (4). They are host specific and can only infect and replicate within specific bacteria. This allows them to target pathogens commonly found in food without reducing the number of commensal bacteria. Phage mediated [biocontrol] of food borne bacteria is not only an effective means treating pathogenic infections, it is also a solution to the fast-emerging antibiotic resistant bacterial strains (5).
Physical environment
Distribution of Phages
Phages are widely distributed in the environment and represent part of the natural microbiological flora of foods (9). A study used to identify [Salmonella]-specific phages isolated a total of 232 phages from 26 sampling sites which included broiler farms, poultry abattoirs, and wastewater plants (3). Bacteriophages which target [Escherichia]. coli are commonly present in sewage, hospital waste water, polluted rivers and fecal samples (7). E. coli phages have been recovered from fresh chicken, pork, ground beef, mushrooms, lettuce and other raw vegetables (1). [Listeria], the bacteria which causes listeriosis is also found on various retail foods and is ubiquitous in the environment (6).
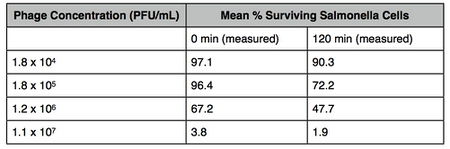
Bacteria to Phage Ratio
Based on kinetics, thermal motion-driven particle diffusion and mixing due to fluid flow or bacterial swimming will result in a higher rate of phage and bacterium encounters (1). When treating solid food, a sufficiently high number of phages is required to infect low numbers of bacteria due to the unlikelihood that they will come into contact.
The concentration threshold of phage numbers is also a factor in reducing the number of bacteria. A study which showed the mean % of surviving Salmonella cells in a nutrient broth decreased significantly with an increase in phage concentration. After 120 minutes, 90.3 % of cells remained when 1.8x10^4 PFU/mL of phage was added, compared to the 1.9% of cells that survived when 1.1x10^7 PFU/mL of phage was added (10). However, if the number of target bacteria falls below a minimum number, the large number of phage required may render phage therapy impractical (3).
Interaction Surfaces
Phages must be applied and retained near the treated surfaces in order to avoid wasteful phage washing and potential inactivation of virulence particles, particularly from wash fluids that clean processing areas (8). Phages need to be immobilized in the right orientation in order to obtain high capture efficacy of bacteria and reduce binding to non-specific bacteria. Through electrostatic interactions, studies have shown that negatively charged phages immobilized to positively charged cellulose membranes resulted in a lowered bacterial count for both Listeria and E. coli O157:H7 studies (8).
Applications in the Food Industry
Treatment Methods
US Food and Drug Administration have recently approved commercial phage preparations to prevent bacterial contamination of livestock, food crops, meat and other foods (4). The actual application strategies can include dipping, spraying, or adding phages as a liquid to a larger volume of food material (1). The timing of phage application should also be considered with regards to the relative point of slaughter and/or packaging (1).
Benefits
Compared to antibiotic treatment, phage mediated biocontrol only infect the targeted subset of bacteria, avoiding the imbalance of commensal flora often caused by broad-spectrum antibiotics. Phages are also self-limiting because they only replicate as long as the targeted bacterium is present (1). Phages are also constantly evolving to circumvent their host’s defenses and resistant bacteria are often less fit than their phage-sensitive counterparts (3).
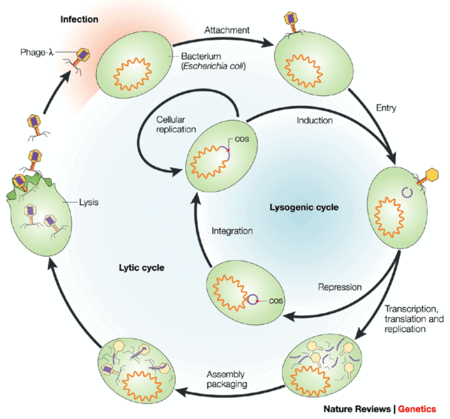
Safety
Studies have show that E. coli phages have no significant effect on the E. coli found in the gut of mice and humans. A reason for this may be due to the fact that commensal E. coli found in test subjects live in niches not easily accessible to environmental phages. Because phages are present in a wide variety of foods consumed every day, it is clear that they are non-toxic to humans (1). There are concerns regarding temperate (lysogenic) phages that do not kill their hosts immediately, and can alter the host’s phenotype through genome integration (1). Most phage genes should be silent, however certain phage virulence toxin genes can otherwise transform harmless bacteria into pathogens (2). Therefore, the selection of an appropriate phage for the biocontrol of pathogenic bacteria is crucial.
Key Microorganisms
E. coli
The World Health Organization estimates that 5 million children die each year as a consequence of acute diarrhea, with E. coli being the cause of a third of the cases in developing countries. E. coli O157:H7 is found in the feces of 7% of cattle at slaughter, and this becomes the source for meat contamination (1)(7). Thus, phage therapy will be most effective in the biocontrol of meat production if used prior to slaughter.
Studies have shown that when is beef artificially contaminated with E. coli with a cocktail of three different phages, no viable bacteria were found in 7 out of 9 samples. Another study has shown the elimination of 94 - 100% of E.coli at higher phage doses on a variety of food products with hard surfaces (1).
Listeria monocytogenes
L. monocytogenes is an opportunistic food borne pathogen that affects the young, the old, immunocompromised and the pregnant women population (1). It causes a serious disease called Listeriosis, with ill-effects ranging from gastroenteritis to septicaemia (11). It can tolerate high levels of salt content, can grow at acidic pH values and can still replicate at temperatures below 1 degrees celsius (9). L. monocytogenes is usually killed during pasteurization or other heat treatments (1), however it has been isolated from ready-to-eat foods such as milk, cheese, cold-cut meats, smoked fish, seafood and vegetables (9). No clear reservoir has been identified, but contaminations can occur via ingredients, factory workers and equipment (1).
Studies have shown that cocktails of different L. monocytogenes phages caused a reduction of 2 to 4.6 logs of bacteria on apple slices (1). The addition of broad-host-range phages such as A511 and P100 to liquid foods caused bacterial counts to drop rapidly below levels of detection. On solid foods, these phages reduced L. monocytogene counts by up to 5 log units (1). In 2006, the US Food and Drug Administration approved the use of phage cocktail for use in ready-to-eat meats to prevent L. monocytogenes contamination (4).
Salmonella
Salmonella causes diarrhea and is one of the principal causes of food-borne illness on a global scale (1). Birds, particularly chicken remains to be the main reservoir by which this bacteria enters the food production system, however it is not the only reservoir, thus other food products can be contaminated (1)[3]. Phage therapy would not introduce new biological agent into the food chain since the Salmonella phage is readily isolated from poultry (3). Because contamination of livestock is the main cause of Salmonella diseases, phage intervention should occur at the pre-slaughter level and post-slaughter level (1).
Studies have shown that bacteriophages can reduce cecal colonization of S. enterica in commercial broiler chickens (3). In post-slaughter treatment, the Salmonella phage Felix O1 caused a 2 log reduction of bacteria in hot dogs (1). The US Environmental Protection Agency approved use of a Salmonella phage product to be sprayed or used as a wash on chicken prior to slaughter (1).
Current Research
Bacterial Resistance to Phage Therapy
Bacteria and their bacteriophage are constantly co-evolving. A study showed that E. coli O157, when incubated with phage PP01 for 200 hours, developed a series of mutants which differed in colony morphology, nature of phage receptors OmpC and LPS, and phage susceptibility (7). The phage responded by evolving a broadened host range (7). A trade off was observed between resistance to phage and competitiveness with parental strains for resources. For phage resistant strains to be selected for in the wild, they must also compete with many other strains that do not feel this phage pressure (unlike competing again only the phage-susceptible ancestor in the laboratory) (7). If phage selective pressure is low, such mutants cannot be expected to present any danger in long-term phage based intervention (1). Depending on the phage however, many bacteria are favoured in this co-evolutionary arms race (some resistance in certain strains even come without a metabolic cost) (7), thus bacterial resistance may still pose to be a problem in the future.
Commercial Production of Phages
In order for phages to be effective in phage-mediated biocontrol, studies must be tested under conditions which resemble commercial practices. For zoonotic bacteria such as Salmonella, there is need to determine the optimal timing and delivery of bacteriophage in a real-life poultry industry setting (3). In order to have this intervention be scaled up for commercial production, cost-effectiveness vs. efficacy in real-life application will need to be assessed (1). Market acceptance by the food industry and the consumer will need to occur before it can be considered an ideal antibacterial agent (1).
References
(1) Hagens, S., Loessner, M.J. “Bacteriophage for Biocontrol of Foodborne Pathogens: Calculations and Considerations.” Current Pharmaceutical Biotechnology 2010, 11, 58-68
(2) Goodridge, L., Abedon, S.T. “Bacteriophage biocontrol and bioprocessing: Application of phage therapy to industry.” Cienciava.pt 2003, 53 (6), 254-262
(3) Atterbury, R.J., Van Bergen, M.A.P., Ortiz, F., Lovell, M.A., Harris, J.A., De Boer, A., Wagenaar, J.A., Allen, V.M., Barrow, P.A. “Bacteriophage Therapy to Reduce Salmonella Colonization of Broiler Chickens.” Appl. Environ. Microbiol 2007, 73 (14), 4543-4549
(4) O’Flaherty, S., Paul Ross, R., Coffey, A. “Bacteriophage and their lysins for elimination of infectious bacteria.” Federation of European Microbiological Societies 2009, 33, 801-819
(5) Teuber, M. “Spread of antibiotic resistance with food-borne pathogens.” Cellular and Molecular Life Sciences 1999, 56, 755-763
(6) Walsh, D., Duffy, G., Sheridan, J.J., Blair, I.S., McDowell, D.A. “Antibiotic resistance among Listeria, including Listeria monocytogenes, in retail foods.” Journal of Applied Microbiology 2001, 90, 517-522
(7) Brussow, H. “Phage therapy: the Escherichia coli experience.” Microbiology 2005, 151, 2133-2140
(8) Anany, H., Chen, W., Pelton, R., Griffiths, M.W. “Biocontrol of Listeria monocytogenes and Escherichia coli O157:H7 in Meat by Using Phages Immobilized on Modified Cellulose Membranes.” Appl. Environ. Microbiol. 2011, 77 (18), 6379-6387
(9) Guenther, S., Huwyler, D., Richard, S., Loessner, M.J. “Virulent Bacteriophage for Efficient Biocontrol of Listeria monocytogenes in Ready-To-Eat Foods.” Appl. Environ. Microbiol. 2008, 75 (1), 93-100
(10) Bigwood, T., Hudson, J.A., Billington, C. “Influence of host and bacteriophage concentrations on the inactivation of food-borne pathogenic bacteria by two phage.” Federation of European Microbiological Societies 2009, 291, 59-64
(11) Allerberger, F., Wagner, M. “Listeriosis: a resurgent foodborne infection.” Austrian Agency for Health and Food Safety 2009, 16, 16-23
Edited by student of Angela Kent at the University of Illinois at Urbana-Champaign.