Pneumococcal meningitis and the role of Streptococcus pneumoniae
Introduction
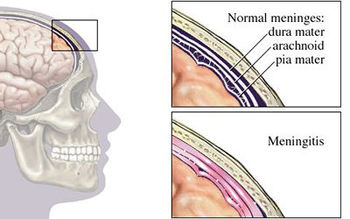
By Avery Anderson
Bacterial meningitis is a potentially life-threatening disease of the central nervous system (CNS). It is characterized by inflammation of the membranes surrounding the brain and spinal cord, known as the meninges. The meninges include the pia mater, the arachnoid and the dura mater that encase and protect the brain and spinal cord. Figure 1 demonstrates the location of the meninges and shows a visual representation of inflammation that occurs during meningitis.
The two leading causes of bacterial meningitis are Streptococcus pneumonia and Neisseria meningitidis [27]. Bacterial meningitis caused by Neisseria meningitidis is known as meningococcal meningitis while bacterial meningitis caused by Streptococcus pneumoniae is referred to as pneumococcal meningitis. Streptococcus pneumoniae accounts for two-thirds of all meningitis cases in the United States and Western Europe [1] and has the highest mortality rate of all other microorganisms causing meningitis [26]. In regions of the world where resources are scarce, fatality rates of pneumococcal meningitis near 51% and even in developed countries the rates are as high as 37% [7].
Streptococcus pneumoniae
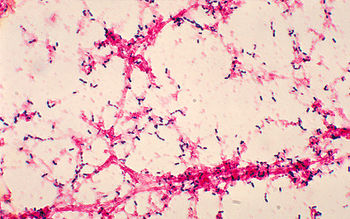
Streptococcus pneumoniae, also referred to as pneumococcus, is a Gram-positive bacteria that belongs to the Firmicutes phylum. Figure 2 shows gram-stained S. pneumoniae grown on blood agar which is the frequent medium used in laboratories when researching this microorganism. It is a non-spore forming and non-motile bacteria [33]. Streptococcus pneumoniae most frequently are diplococci in structure, meaning they occur in pairs, but they can also exist individually or in short chains. Each individual cell measures between 0.5 and 1.25 micrometers in diameter [34]. Its optimal growth environment is 35-37 degrees Celsius [10]. This microorganism contains a circular genome with over 2 million base pairs [36]. Different strains of the bacteria can vary up to 10% in genetic information, but are consistent with a reoccurring set of 1553 genes [36]. Pneumococci are facultative anaerobes having the flexibility to ferment glucose into lactic acid for energy or utilize aerobic respiration for energy depending on the amount of oxygen available in its environment [33]. S. pneumoniae has the ability to oxidize hemoglobin characterizing it as an alpha-hemolytic bacteria. In the laboratory, the pneumococci's alpha-hemolytic property is helpful for researchers to identify it because it appears green on blood agar from its ability to induce hemolysis [34].
Streptococcus pneumoniae can live naturally in the human nasopharynx without causing disease. However, commensal carriage rates directly affect the transmission of S. pneumoniae [32] within any given population. Meningitis is sometimes said to be community-acquired because the carriage rates of S. pneumoniae increase in crowded situations. For example, children, who typically have the highest carriage rates nearing 37%, in crowded environments like that of day care centers have carriage rates that can reach as high as 58% [27]. Likewise, 50-60% of adult service personnel on military bases are likely to be carriers compared to the 5-10% of all healthy adults without children [11].
Pathogenic S. pneumoniae are encapsulated in polysaccharides whereas commensal S. pneumoniae do not have the same capsule. This capsule proves to be important to the virulence of the microorganism [11] in evading the human immune responses. When pathogenic, S. pneumoniae is responsible for pneumococcal disease, which includes meningitis, pneumonia, parapneumonic empyema, occult bacteremia, sepsis, arthritis, peritonitis, and endophthalmitis [3]. Transmission of S. pneumoniae most often occurs through respiratory droplets, in other words coughing or sneezing [11], making the means of contracting pneumococcal disease dangerously easy.
Pneumococci has over 93 known serotypes that are differentiated based on the composition of capsular polysaccharide [9]. Only a few of these serotypes account for the majority of disease causing agents worldwide including serotypes 1, 5, 6A, 6B, 14, 19F, and 23F [20]. The serotype responsible for pneumococcal meningitis outbreaks in the African meningitis belt is serotype 1 [20].
Pneumococcal Meningitis Pathogenesis
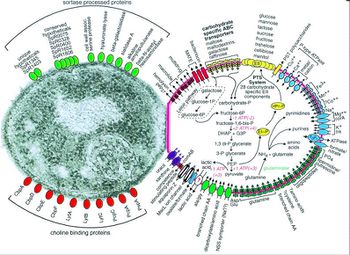
Before Streptococcus pneumoniae has the chance to ruminate as pneumococcal meningitis in the brain and spinal cord it must first find a way to colonize in the nasopharynx mucus, invade the mucosal epithelium, survive in the bloodstream, and traverse the blood-brain barrier [21]. Streptococcus pneumoniae are estimated to possess over 500 surface proteins that allow for interaction between the microorganism and the host [21]. The genes encoding these surface proteins are known as virulence genes because they are largely responsible for the microorganisms ability to infect and cause disease in the host [32]. Of these surface proteins, choline-binding proteins are especially important in agitating the inflammatory response in the brain that is characteristic of meningitis. Many of the surface proteins are depicted in Figure 3.
One of the first human defenses against S. pneumoniae involves respiratory mucus that physically block the bacteria from adhering to the epithelial cell layer and lysozymes, which are enzymes that catalyze the break down of the bacterial cell wall [29]. S. pneumoniae is able to evade these defenses by its expression of enzymes (NanA, BgaA, StrH, and NanB) that decrease mucus viscosity (shown in Figure 4), thus increasing the difficulty for the mucus layer to entrap the bacteria. Additionally, the negative charge of the pneumococci capsule allows it to repel from the sialic acid of the mucus further evading entrapment [27]. To escape destruction by lysozymes, S. pneumoniae expresses two enzymes, peptidoglycan N-acetylglucosamine-deacetylase A (PdgA) and O-acetyltransferase (Adr), that modify the peptidoglycan consequently inhibiting lysozyme cleavage [14] (Figure 4).
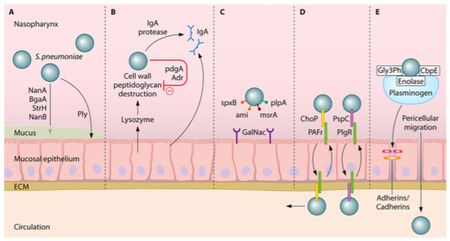
Immunoglobulin A (IgA), an antibody released by the human body for immunity, is unable to bind to the S. pneumoniae because of the polysaccharide capsule and the pathogen is able to cleave IgA with IgA protease, as demonstrated in Figure 4 [27]. One of the surface proteins on the pneumococci, surface protein A (PspA), binds lactoferrin, a immune system protein with antimicrobial properties, preventing its bactericidal activity. Pneumococci have the advantageous ability to manipulate the binding properties of its capsule through phase variation, adjusting the polysaccharide composition of the capsule [27]. To evade the initial immune defenses, the pneumococcal capsule is thick, opaque, to protect it against entrapment by mucus or breakdown from immunoglobulin and complement binding [18]. Following survival of those mechanisms, the capsule is thin, transparent, exposing adhesion molecules to interact with the host nasopharyngeal epithelium, the necessary step to gain entry to the bloodstream [37].
The microorganism utilizes its surface proteins, namely the phosphorylcholine to bind to the platelet activating factor (PAF) receptor and choline-binding protein PspC, and binds to glycoconjugates on the epithelium (Figure 4) to facilitate cell transcytosis, crossing through the epithelium cells into the bloodstream [27]. Surviving immune defenses in the bloodstream requires the protection of the polysaccharide capsule and binding of surface proteins to complement components. Pneumolysin is a virulence factor released by S. pneumoniae that allows the microorangism to escape phagocytosis by decreasing complement factors [2].
Similarly to the transcytosis process of the epithelium cells, S. pneumoniae in the transparent capsule variation binds phosphoylcholine to PAF receptors of endothelial cells. Pneumococcal surface protein C (PspC) additionally binds to laminin receptors on the endothelial cell facilitating S. pneumoniae entry into cerebral spinal fluid [19].
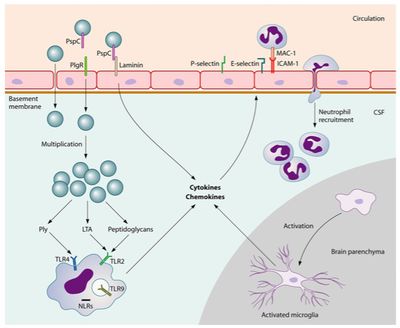
There is a lot of interest surrounding the mechanisms in which pneumococci are able to bind to the cerebral endothelium and penetrate the blood brain barrier (BBB). In a experiment conducted by Orihuela et al. (2009), researchers took a closer look at how binding of the pathogen takes place. First, pneumococci binding to immobilized recombinant laminin receptors (LR) was established through ELISA. Mouse, rat, and human endothelial cells containing surface laminin receptors were then analyzed for bacterial adherence. The expression of LR was found to be associated with much higher levels of pneumococci adherence than when laminin, binds to a domain of the LR, or an antibody against the C terminus of the LR were present [30].
Knowing that PspC is necessary for S. pneumoniae to bind to the BBB from previous research, Orihuela and colleagues (2009) used affinity chromatography with matrix-assisted laser desorption/ionization time-of-flight analysis to determine 37/67-kDa LR as the specific ligand. After further analysis, researchers were able to reveal that PspC deletion mutants demonstrated significantly less bacterial adherence. Likewise, when a model of pneumococcal meningitis was established in mice and used to test the functional implications of bacterial adherence, researchers saw decreased instances of disease. PspC mutant mice, in which only two amino acids were manipulated to disable binding to the carboxyl terminus of the LR, developed pneumococcal meningitis significantly less than wild-type mice [30]. The binding of pneumococci to a laminin receptor is depicted in Figure 5.
Disruption of tight junctions between endothelial cells also appears to be necessary for the disease to translocate across the blood-brain barrier [19]. When the pneumococci reach the cerebral spinal fluid they are able to multiply freely. Pneumococci binding to pattern recognition receptors sets off a cascade of inflammatory reactions initiated by the release of cytokines from antigen-presenting cells (APCs) [27]. This process involves the recruitment of white blood cells, including neutrophils and lymphocytes, from the blood to the region of S. pneumoniae occupation [27]. The APCs are present in various locations in the brain including the cerebral spinal fluid, meninges, choroid plexus, or perivascular space [13]. Stimulating the pattern recognition receptors of ACPs up regulates the production of cytokines, expressing a pro-inflammatory response [27]. Leukocytes are attracted into the cerebral spinal fluid by pro-inflammatory cytokines as well as cerebral perivascular and meningeal macrophages [27]. The following increase in release of reactive oxygen species and reactive nitrogen species leads to destabilizing oxidative stress in the brain [15].
Interaction with other microbial species
The survival of S. pneumoniae in the nasopharynx is in some part dependent on the other microbial species present there as well. Prior exposure to the influenza virus makes the host increasingly vulnerable to pathogenic pneumococci [27]. Interestingly, studies examining the competitive colonization of S. pneumoniae and Haemophilus influenza revealed phagocytosis, and complete bacterial clearance, of S. pneumoniae stimulated by H. influenza [24]. Though the reasons are unknown, epidemiological data demonstrated a negative correlation between Staphylococcus aureus and S. pneumoniae in nasopharyngeal colonization [8]. A potential mechanism of competitive colonization and outgrowth could be the production of pneumocins, small peptides that can kill other related microbial species, by S. pneumoniae [31]. Killing other microorganisms also allows S. pneumoniae the opportunity to pick up foreign DNA and integrate it into its own genome so that it might obtain a survival advantage [23].
Symptoms & Diagnosis
Pneumococcal meningitis might be difficult to detect in the early stages of the disease because symptoms may be common among other illnesses. For instance, fever, chills, nausea and vomiting are all symptoms of meningitis in addition to many other ailments [35]. Rash and stiff neck are often referenced as the distinctive symptoms of meningitis, though pneumococcal meningitis patients often do not develop the typical rash seen in meningococcal meningitis patients [25]. Other symptoms include increased heart rate, changed mental status, sever headache, and rapid breathing. [35]. When symptoms occur it is important to address them immediately as survival can be dependent on how quickly the disease is recognized and treated. A study examining the cases of nearly 700 pneumococcal meningitis patients reports the highest occurring symptoms to consist of headache (87% of patients), fever (86%), neck stiffness (81%), and change in mental status (81%) [17]. About 20% of patients diagnosed with pneumococcal meningitis will die from it and up to 50% of patients will suffer from some neurological sequelae [22].
Pneumococcal meningitis is considered an emergency because it is one of the most life-threatening infectious diseases of the CNS [4]. If a doctor is suspicious of pneumococcal meningitis a blood sample and/or lumbar puncture will be ordered for medical diagnosis. The lumbar puncture consists of sampling the patient’s cerebral spinal fluid. The sample is then cultured, usually on blood agar plates [10]. Blood tests to assess immune activity of the patient may also be useful to the physician for better understanding the stage of progression for the disease.
Risk Factors
There are a number of risk factors for pneumococcal meningitis including age, environment, and medical history. With the use of conjugated vaccines for young children, the age that is now most affected by pneumococcal meningitis is the elderly. Most patients in the Netherlands have an average age of 50 [1]. It is often more difficult to identify meningitis in the elderly because it does not present the same way it does in younger individuals. Focal neurological abnormalities are seen more often than the notable stiff neck or headache [7].
Since Streptococcus pneumoniae can inhabit the nasopharynx asymptomatically any agent causing the immune system to be compromised will make an individual more susceptible to contraction of pneumococcal meningitis. Diabetes mellitus patients are often more vulnerable to infection. In fact, in 7-10% of adults cases of community-acquired bacterial meningitis diabetes was a preexisting disease [1]. Alcoholism is another disease that increases the likelihood of contracting bacterial meningitis potentially because of the suppressed immune response [39]. When compared to nonalcoholic patients with bacterial meningitis, alcoholics experienced greater rates of complications and worse outcomes [1]. Another risk factor includes having undergone a splenectomy. The spleen plays an important function in clearing bacteria from the bloodstream so without it the body becomes particularly prone to infection [1]. Another risk factor especially vulnerable to pneumococcal meningitis is HIV infection due to the depreciated immune response. If an individual is previously diagnosed with HIV/AIDS, then his/her susceptibility to pneumococcal disease is increased anywhere from 6- to 324-fold [7]. Other factors that may be cause for greater chances of developing pneumococcal meningitis include having cancer, genetic susceptibility or anatomical defects [1].
Neurological sequelae
Up to 35% of surviving patients come to incur at least one sequelae such as cognitive deficits, hearing loss, motor deficits, seizures, visual impairment and hydrocephaly [16]. Much of the neurological damage resulting from pneumococcal meningitis is a consequence of the host’s immune response. Microglial cells that become activated when pneumococci binds to pattern recognition receptors increase production of cytokines, reactive oxygen species and reactive nitrogen species that are intended to be neuroprotective but in large amounts prove to be neurotoxic [27]. Some of the various causes of neurological damage include increased intracranial pressure, cytotoxic and vasogenic edema, herniation, leukocyte infiltration, abscess formation, cortical necrosis and hippocampal neuronal loss [27]. Figure 6 shows images from pneumococcal meningitis patients with lasting neuronal damage. S. pneumoniae additionally produces hydrogen peroxide and pneumolysin which act as neurotoxins and initiate cell death of neurons, microglial and brain microvascular endothelial cells [6]. Pneumococci induced neuronal apoptosis is seen to appear largely in the dentate gyrus of the hippocampus [6].
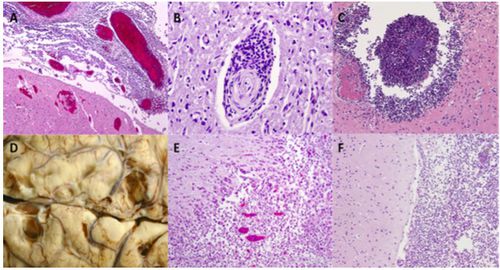
Hearing loss is one of the common neurological deficits occurring in pneumococcal meningitis survivors. Up to 30% of patients endure either uni- or bilateral cochlear damage resulting in frequently severe hearing loss [40]. When S. pneumoniae reach the cochlear perilymphatic system severe granulocytic inflammation of the region occurs [5]. A study analyzing pneumococcal isolates found that serotype 3 is associated with the greatest prevalence of resulting hearing loss [17].
Treatment & Adjunctive Therapies
The primary treatment of pneumococcal meningitis is antibiotic administration. One of the most commonly prescribe drugs is Ceftriaxone [34]. Vancomycin, penicillin, and other antibiotics are also used depending on specific susceptibility of S. pneumoniae present [38]. S. pneumoniae can develop resistance to penicillin and drug resistance is becoming more and more prevalent in patient cases; thus, doctors may need to use other antibiotics or a combination of antibiotics to effectively treat the disease [38]. More research into antibiotic discovery will help open up alternative avenues of treatment and be especially helpful to patients with antibiotic resistance strains of pneumococci.
Vaccines are also available for certain strains of the bacteria. The type of vaccine necessary may be dependent on age and medical history. Two commonly recommended vaccines for the adult population include a pneumococcal conjugate vaccine (PCV13) and a pneumococcal polysaccharide vaccine (PPSV23) [28]. PCV13 is also the vaccine most frequently recommended for all children 5 years old and younger. Individuals that are at high risk for developing pneumococcal disease typically are recommended to receive the PPSV23 vaccine [12].
There are several mechanisms and processes in the human body that S. pneumoniae has to survive before culminating in blood-brain barrier traversal and ultimately as pneumococcal meningitis. Each of these steps is a potential avenue for adjunctive therapy.
When S. pneumoniae is recognized in the body through binding to Toll-like receptors (TLRs), receptors that are meant to tag exogenous invaders and activate immune response, it initiates a cascade of reactions that lead to the release of molecules for a pro-inflammatory response [4]. This response includes the expression of cytokines, chemokines, and other pro-inflammatory molecules in the body. This reaction is meant to attack the pathogen, but the extent of inflammation actually becomes damaging to the brain [4]. Inhibition of this pro-inflammatory response by cytokines is a target for treatment, but more research is needed to determine what is a healthy level of cytokines that can fight off the microorganism while minimizing the harmful effects of inflammation in and around the brain [4].
The release of cytokines is also associated with the activation of matrix metalloproteinases (MMPs), which increase the permeability, and thus vulnerability, of the blood-brain barrier by breaking down the extracellular matrix and myelin proteins [4]. Inhibiting the activity of MMPs has been shown to limit the degradation of the blood-brain barrier in the hippocampus and the cortex [4].
A corticosteroid treatment with dexamethasone has been tested as a therapeutic method, but the results have not been unequivocally positive. In an experimental model of pneumococcal meningitis in adult rats, dexamethasone treatment prevented cognitive impairment but led to greater oxidative stress in the brain [4]. Animal trials with experimental models of pneumococcal meningitis in rabbits and gerbils have shown reductions in hearing loss when administered dexamethasone treatment; however, results were less effective in infant animals [27]. Though some trials have shown results with minimal effects of dexamethasone, it has become routine therapy in many countries for individuals who suspect to have or have been diagnosed with pneumococcal meningitis [27].
Another approach to treatment involves cannabidiol, a non-psychotropic cannabinoid component that demonstrates neuroprotective activity in addition anti-inflammatory, antioxidant, and analgesic effects [4]. When tested in rats subjected to pneumococcal meningitis, cannabidiol treatment appeared to protect against memory impairments [4].
Glycerol treatment is an additional method of explorative treatment. Glycerol is generally inexpensive and easily accessible making it a viable option for pneumococcal patients. It’s expected that increased glycerol will show improvements in blood flow to the brain and thus oxygenation of the brain. This result may potentially help attenuate some of the neurological sequelae symptoms exhibited in surviving meningitis patients [27]. Clinical trials have not found definitive answers to whether this treatment is effective and safe. A study including children with meningitis saw decreased rates of hearing loss after glycerol administration; however, another research group found glycerol treatment to be associated with increased hippocampal neuronal apoptosis in experimental models of pneumococcal meningitis in rats [27].
Other adjunctive treatments have been explored including inhibition of caspase activation, tryptophan pathway inhibition, mesenchymal stem cells, and other alternatives [4]. More research is necessary to better understand techniques that can attenuate the neurological deficits associated with pneumococcal meningitis.
Conclusion
Pneumococcal meningitis is caused by bacterial infection of the CNS from S. pneumoniae. Though the disease can be severe and life-threatening, there are a number of antibiotic treatment options. Vaccinations have also played a large role in reducing the prevalence of this disease. Regardless of medical advances however, mortality caused by pneumococcal meningitis continues to vary up to 37% and neurological sequelae can affect up to 52% of surviving patients [27]. Further research can help reveal methods of attenuating lasting neurological deficits that are often associated with the disease. Children and elderly should be especially cognizant of the signs and symptoms of pneumococcal meningitis and contact a physician if he/she suspects possible infection.
References
[1] Adriani, K. S., Brouwer, M. C., & van de Beek, D. (2015). Risk factors for community-acquired bacterial meningitis in adults. The Netherlands Journal of Medicine. 73: 53-60.
[2] Alcantara, R. B., Preheim, L. C., & Gentry-Nielsen, M. J. (2001). Pneumolysin-induced complement depletion during experimental pneumococcal bacteremia. Infect. Immun. 69: 3569-3575.
[3] Alsina, L., Basteiro, M. G., de Paz, H. D., Inigo, M., de Sevilla, M. F., Trivino, M., Juan, M., & Munoz-Almagro, C. (2015). Recurrent invasive pneumococcal disease in children: underlying clinical conditions, and immunological and microbiological characteristics. PLoS ONE. 10: 1-13.
[4] Barichello, T., Collodel, A., Generoso, J. S., Simoes, L. R., Moreira, A. P., Ceretta, R. A., Petronilho, F., & Quevedo, J. (2015). Targets for adjunctive therapy in pneumococcal meningitis. J. Neuroimmunol. 278: 262-270.
[5] Brandt, C. T., Caye-Thomasen, P., Lund, S. P., Worsoe, L., Ostergaard, C., Frimodt-Moller, N., Espersen, F., Thomsen, J., & Lundgren, J. D. (2006). Hearing loss and cochlear damage in experimental pneumococcal meningitis, with special reference to the role of neutrophil granulocytes. Neurobiol. Dis. 23: 300–311.
[6] Braun, J. S., Sublett, J. E., Freyer, D., Mitchell, T. J., Cleveland J. L., Tuomanen, E. I., & Weber, J. R. (2002). Pneumococcal pneumolysin and H(2)O(2) mediate brain cell apoptosis during meningitis. J. Clin. Invest. 109: 19-27.
[7] Brouwer, M. C., Tunkel, A. R., & van de Beek, D. (2010). Epidemiology, diagnosis, and antimicrobial treatment of acute bacterial meningitis. Clin. Microbiol. Rev. 23: 467-492.
[8] Bogaert, D., van Belkum, A., Sluijter, M., Luijendijk, A., de Groot, R., Rumke, H. C., Verbrugh, H. A., & Hermans, P. W. M. (2004). Colonisation by Streptococcus pneumoniae and Staphylococcus aureus in healthy children. Lancet. 363: 1871-1872.
[9] Center for Disease Control and Prevention. (2014). Epidemiology of meningitis caused by Neisseria meningitidis, Streptococcus pneumoniae, and Haemophilus influenza. Web. http://www.cdc.gov/meningitis/lab-manual/chpt02-epi.html
[10] Center for Disease Control and Prevention. (2014). Identification and characterization of Streptococcus pneumoniae. Web. http://www.cdc.gov/meningitis/lab-manual/chpt08-id-characterization-streppneumo.html
[11] Center for Disease Control and Prevention. (2012). Pneumococcal disease: epidemiology and prevention of vaccine-preventable diseases. The Pink Book. Web. http://www.cdc.gov/vaccines/pubs/pinkbook/pneumo.html
[12] Center for Disease Control and Prevention. Pneumococcal Vaccination. Web. http://www.cdc.gov/vaccines/vpd-vac/pneumo/default.htm
[13] Chinnery, H. R., Ruitenberg, M. J., & McMenamin, P. G. (2010). Novel characterization of monocyte-derived cell populations in the meninges and choroid plexus and their rates of replenishment in bone marrow chimeric mice. J. Neuropathol. Exp. Neurol. 69: 896-909.
[14] Davis, K. M., Akinbi, H. T., Standish, A. J., & Weiser, J. N. (2008). Resistance to mucosal lysozyme compensates for the fitness deficit of peptidoglycan modifications by Streptococcus pneumoniae. PLoS Pathog. 4:e1000241
[15] de Menezes, C. C., Dorneles, A. G., Sperotto, R. L., Duarte, M. M. F., Schetinger, M. R. C., & Loro, V. L. (2009). Oxidative stress in cerebrospinal fluid of patients with aseptic and bacterial meningitis. Neurochem. Res. 34: 1255-1260.
[16] Edmond, K., Clark, A., Korczak, V. S., Sanderson, C., Griffiths, U. K., & Rudan, I. (2010). Global and regional risk of disabling sequelae from bacterial meningitis: a systematic review and meta-analysis. Lancet Infect. Dis. 10: 317-328.
[17] Heckenberg, S. G. B., Brouwer, M. C., van der Ende, A., Hensen, E. F., & van de Beek, D. (2012). Hearing loss in adults surviving pneumococcal meningitis is associated with otitis and pneumococcal serotype. Clin. Microbiol. Infect. 18: 849-855.
[18] Hyams, C., Camberlein, E., Cohen, J. M., Bax. K., & Brown, J. S. (2010). The Streptococcus pneumoniae capsule inhibits complement activity and neutrophil phagocytosis by multiple mechanisms. Infect. Immun. 78: 704-715.
[19] Iovino, F., Orihuela, C. J., Moorlag, H. E., Molema, G., & Bijlsma, J. J. (2013). Interactions between blood-borne Streptococcus pneumoniae and the blood-brain barrier preceding meningitis. PLoS ONE. 8: e68408.
[20] Johnson, H. L., Deloria-Knoll, M., Levine, O. S., Stoszek, S. K., Hance, L. F., Reithinger, R., Muenz, L. R., & O’Brien, K. L. (2010). Systematic evaluation of serotypes causing invasive pneumococcal disease among children under five: the pneumococcal global serotype project. PLoS Medicine. 7: 1-13.
[21] Koedel, U., Scheld, W. M., & Pfister, H.-W. (2002). Pathogenesis and pathophysiology of pneumococcal meningitis. Lancet Infect. Dis. 2: 721-736.
[22] Liechti, F. D., Grandgirard, D., & Leib, S. L. (2015). The antidepressant Fluoxetine protects the hippocampus from brain damage in experimental pneumococcal meningitis. Neuroscience.
[23] Lux, T., Nuhn, M., Hakenbeck, R., & Reichmann, P. (2007). Diversity of bacteriocins and activity spectrum in Streptococcus pneumoniae. J. Bacteriol. 189: 7741-7751.
[24] Lysenko, E. S., Ratner, A. J., Nelson, A. L., & Weiser, J. N. (2005). The role of innate immune responses in the outcome of interspecies competition for colonization of mucosal surfaces. PLoS Pathog. 1: e1.
[25] Meningitis Research Foundation. Pneumococcal meningitis. Web. http://www.meningitis.org/disease-info/types-causes/pneumococcal
[26] Mitchell, A. J., Yau, B., McQuillan, J. A., Ball, H. J., Too, L. K., Abtin, A., Hertzog, P., Leib, S. L., Jones, C. A., Gerega, S. K., Weninger, W., & Hunt, N. H. (2012). Inflammasome-dependent IFN-y drives pathogenesis in Streptococcus pneumoniae meningitis. The Journal of Immunology. 189: 4970-4980.
[27] Mook-Kanamori, B. B., Geldhoff, M., van der Poll, T., & van de Beek, D. (2011). Pathogenesis and pathophysiology of pneumococcal meningitis. Clin. Microbiol. Rev. 24: 557-591.
[28] National Foundation for Infectious Diseases. Adult Vaccinations: Pneumococcal Disease. Web. http://www.adultvaccination.org/pneumococcal_vaccine_vaccination_adult_immunization.htm
[29] Nelson, A. L., Roche, A. M., Gould, J. M., Chim, K., Ratner, A. J., & Weiser, J. N. (2007). Capsule enhances pneumococcal colonization by limiting mucus-mediated clearance. Infect. Immun. 75: 83-90.
[30] Orihuela, C. J., Mahdavi, J., Thornton, J., Mann, B., Wooldridge, K. G., Abouseada, N., Oldfield, N. J., Self, T., Ala'Aldeen, D. A. A., & Tuomanen, E. I. (2009). J. Clin. Invest. 19: 1638-1546.
[31] Preston, J. A., & Dockrell, D. H. (2008). Virulence factors in pneumococcal respiratory pathogenesis. Future Microbiol. 3: 205-221.
[32] Regev-Yochay, G., Raz, M., Dagan, R., Porat, N., Shainberg, B., Pinco, E., Keller, N., & Rubinstein, E. (2004). Nasopharyngeal carriage of Streptococcus pneumoniae by adults and children in community and family settings. Clin. Infect. Dis. 38: 632-639.
[33] Slonczewski & Foster (2014) Microbiology: An Evolving Science (Norton, 3E).
[34] Todar, K. (2003). Online textbook of bacteriology. Web. http://textbookofbacteriology.net/S.pneumoniae.html
[35] U.S. National Library of Medicine. Meningitis - pneumococcal. Medline Plus. (2012) Web. http://www.nlm.nih.gov/medlineplus/ency/article/000607.htm
[36] van der Poll, T., & Opal, S. M. (2009). Pathogenesis, treatment, and prevention of pneumococcal pneumonia. Lancet 374: 1543–1556.
[37] Weber, J. R., & Tuomanen, E. I. (2007). Cellular damage in bacterial meningitis: an interplay of bacterial and host driven toxicity. J. Neuroimmunol. 184: 45-52.
[38] WebMD. (2012). Antibiotics for bacterial meningitis. Web. http://www.webmd.com/brain/antibiotics-for-bacterial-meningitis
[39] Weisfelt, M., de Gans, J., van der Ende, A., & van de Beek, D. (2010). Community-acquired bacterial meningitis in alcoholic patients. PLoS ONE. 5: e9102.
[40] Weisfelt, M., Hoogman, M., van de Beek, D., de Gans, J., Dreschler, W. A., & Schmand, B. A. (2006). Dexamethasone and long-term outcome in adults with bacterial meningitis. Ann. Neurol. 60: 456–468.
Authored for BIOL 238 Microbiology, taught by Joan Slonczewski, 2015, Kenyon College.