Spoiler Alert: Zygosaccharomyces Rouxii and It's Role in Food Spoilage and Fermentation
Introduction
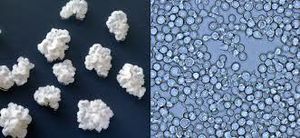
By Daniel Maffezzoli
Zygosaccharomyces rouxii is an interesting species of yeast that is infamous within the food industry. Its capacity to thrive in both highly saline and sugar-dense environments is what makes Z. rouxii a predominant food spoiling agent. The metabolism and ruggedness of the microbe also allow it to resist typical preservative methods in food production. In addition to being a spoiling agent, Z. rouxii is actually used to create various staple ingredients in East Asian cuisine. More specifically, Z. rouxii is the principal microbe used in the fermentation of soybeans during the brewing process of soy sauce, and in the production of miso. This page aims to provide detailed information on the unique metabolism and homeostatic tendencies of this microbe, how the microbe goes about spoiling foods, and the productive qualities that arise from the spoilage-inducing features – particularly in the context of brewing soy sauce.
Habitat, Physiology, and Metabolism
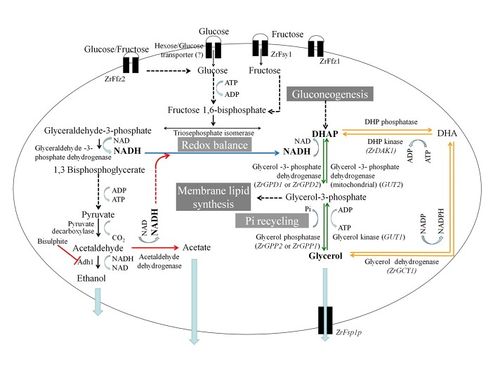
The relationship between habitat and metabolism is typically the most telling aspect of any microbe. The habitat of a given microbe can give large clues about how its metabolism functions, and vice versa. What makes the habitat-metabolism relationship in Z. rouxii so unique is not only that its most ideal growth conditions are extreme by conventional standards, but also that it makes a home in food products that are generally considered to be “non-perishables.” Z. rouxii is the most prominently found microbe in spoiled syrups, honey, fruit juice concentrates, jams, jellies, sauces, sodas, and various shelf-stable condiments like ketchup.[2]
The capacity for Z. rouxii to grow in these microbe-unfriendly environments is a direct result of the osmotolerant and halotolerant properties of its metabolic pathways. It is important to differentiate these characteristics due to the fact that each distinct solute results in a specific corresponding stress response by the microorganism. Z. rouxii is one of the few yeast species that seems to have minimal stress responses to the two conventional environmental stressors of high glucose and high salinity. Researchers have observed Z. rouxii growth in conditions of up to 65%(w/v) D-glucose and 25%(w/v; 4.5M) NaCl which amounts to water activity (aw) values of 0.88 and 0.85, respectively. [3][1] In addition to its osmotic adaptability, Z. rouxii is fructophilic, which allows for more diverse sources of energy. The ability to tolerate such high levels of extracellular solutes and the stressors that accompany them can be traced to the three main regulatory physiological and metabolic pathways in yeasts – the alteration of the cell wall and plasma membrane, changes to the transport systems of the cell, and equilibration of metabolic osmolytes.[1]
The structure of the cell wall and plasma membrane of a yeast cell is integral to its tolerance of high osmotic pressure and salinity. Yeasts are capable of reshaping their morphological composition in order to counter the osmotic pressure of their extracellular environments. The sturdy cell wall of a yeast microbe is comprised of three categories of molecules: mannan, glucan and chitin.[4] Initial research determined that the level of mannans in the Z. rouxii cell wall decreased in the presence of high extracellular NaCl content.[5] The results of studies like these presented a correlation between cell wall thickness and halotolerance, but more recent strain-specific studies determined that certain strains of Z. rouxii with more malleable cell walls were actually more halotolerant than strains with rigid walls.[6] These results clearly suggest a relationship between cell wall mannan and glucan composition and cell wall integrity, although there is further research to be done to reinforce this morphological stress tolerance mechanism. However, the regulatory pathway that induces changes in the cell wall in Z. rouxii has been fairly well researched using S. cerevisiae as a model.[7] In S. cerevisiae the primary pathway for regulating cell wall rigidity is the cell wall integrity signaling pathway (CWISP). Since there is evidence to support the fact that this pathway is rooted in the genetic expression of the yeasts, and Z. rouxii is the Zygosaccharomycete most phylogenically similar to S. cerevisiae, it has been suggested in genetic research that this pathway is likely the one used by Z. rouxii.[7] The CWISP is triggered when osmotic stress is induced. When sensor proteins on the cell wall are activated, signals are sent to the enzyme Rho1 GTPase.[1] This enzyme then triggers the kinesis of multiple effectors which, depending on the character of the initial stimulus, either up- or down-regulate the production and transport of the structural polymers discussed earlier like mannans and glucans to fortify or reduce the cell wall’s integrity. The CWISP has also been observed as a response to mechanical stress stimuli and simple cell wall maintenance.[1]
The second physiological and metabolic pathway that plays a role in the osmotolerance of Z. rouxii is its use and modulation of sugar transporters. Unlike S. cerevisiae, which takes in hexoses by way of facilitated diffusion when in a glucose-depleted hyperosmotic environment, Z. rouxii is a fructophilic microbe and actually prefers a fructose-rich extracellular environment. As pictured in Figure 1, which depicts the variety of metabolic pathways of fructose and glucose that Z. rouxii possesses, the yeast employs two fructose and glucose uptake facilitators when the external levels of those sugars are high. Additionally, researchers in Portugal recently determined that Z. rouxii makes use of a high-affinity, but low-capacity fructose transport protein that is up-regulated when the yeast is in growth medium with low sugar concentration.[2]
The regulation of sugar transporters leads to the final regulatory pathway involved in the high levels of tolerance of Z. rouxii when grown in unfavorable environments, which is the production and sequestration of metabolic osmolytes. The balance of osmolytes is integral to maintaining various homeostatic thresholds like water balance and pH. The principal osmolyte that is used to maintain homeostasis in hyperosmotic environments is glycerol. In general, there are two main metabolic pathways of glycerol that yeasts use (both pictured in Figure 1): the Gcy-Dak pathway and the Gpd-Gpp pathway. The Gpd-Gpp pathway is the primary metabolic pathway utilized by Z. rouxii. In S. cerevisiae, glycerol retention is integral to the maintenance of intracellular water content when responding to osmotic stress. As a result, the cells will biosynthesize large quantities of the molecule, which is inefficient because most of it is lost to the extracellular medium because of the concentration gradient.[8] In Z. rouxii, however, we see something different, which is that the cells will biosynthesize much smaller amounts of glycerol and instead decrease the permeability and pliability of their plasma membranes via manipulated surface phospholipid compositions.[6] This strategy ends up being much more effective as a response to hyperosmotic stress than that of S. cerevisiae and can be connected to the ability of Z. rouxii to survive in hyperosmotic or saline environments.
In addition to hyperosmosis, there are other environmental stress factors that Z. rouxii withstands substantially. The supplemental tables provided at the bottom of the page demonstrate the range of pH, NaCl, sorbate and benzoate levels in which Z. rouxii showed significant positive growth.[9]
Food Spoilage
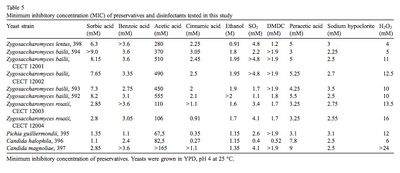
Second only to preventing the presence of pathogenic microbes in food, being able to defend against spoiling agents is an incredibly important part of food production and preservation. Food producers will manipulate and control many aspects of their products to keep the likelihood of contamination by spoilage microbes as low as possible. Factors such as aw, pH, temperature, and many others throughout the production process that are effective on pathogens are also used to kill spoilage microbes like yeasts and fungi. In fact, specific preservatives are often added to combat the spoilage agents. The most commonly used preservatives tend to be weak acids like sorbic, benzoic or propionic acids.[10] These preservatives are especially prevalent in high-sugar foods, but nonetheless not entirely effective. It has been substantially demonstrated in the food science field and in the food production industry that sugar- and salt-tolerant strains of yeasts cause spoilage of mid- or low-moisture food products.[3] As discussed earlier in the page, Z. rouxii has been isolated as a spoiling agent in a variety of high-sugar, low-moisture foods like honey, syrups, etc. The majority of the same strains of yeasts that are resistant to biological or chemical preservative methods are also able to grow in anaerobic conditions making typical mechanical preservation methods like gas packaging susceptible to spoilage (Figure 2).[3] [10] This is obviously problematic to the food industry because it creates a thin line to walk in terms of finding ways to combat resistant strains while maintaining publicly perceived appropriate levels of preservatives in their products.
Soy Sauce Production
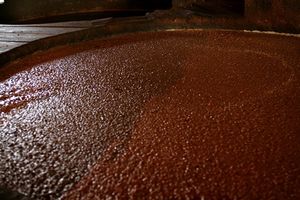
Despite all of the negative press about Z. rouxii and other species of Zygosaccharomycetes, the microbe is actually very productive when utilized in fermentation processes.
The process of brewing the current form of soy sauce has been around for millennia, dating back to 2nd Century China. The production process begins with cooked soybeans and milled wheat, which are then mixed and inoculated with Aspergillus spores (called koji in Japanese), the species of which is typically proprietary to the brewer. The mixture is left to ferment aerobically for a period of about two days. During this time the mold spores flourish and produce extracellular enzymes like amylases and proteases that hydrolyze the starches and proteins in the soy and wheat mixture. Then, a brine solution (22-23% NaCl) is added to set the proper environment for lactic acid bacteria to function. This saline mixture is left to ferment at around 300oC in a semi-aerobic environment. This mixture is called momori (Figure 3) and consists of the brine solution, a cocktail of halotolerant lactic acid bacteria (predominantly Tetragenococcus halophilia), and the mixture of partially broken-down soy and wheat.[11] The lactic acid produced by T. halophilia reduces the pH of the mixture from a neutral 7.0 to about 5.0 before the bacteria can no longer sustain growth. Once it hits this pH threshold, Z. rouxii takes over the fermentation. The yeast proceeds to undergo alcoholic fermentation, which results in a 2% ethanol output. More importantly, however, Z. rouxii produces various flavor compounds including 4-hydroxyfuranones and higher alcohols that are essential characteristics of the taste of soy sauce.[11] This first stage of brine fermentation takes upwards of 7 months to a year. A secondary stage of fermentation occurs with another halotolerant yeast, Candida versitalis, in which final flavor compounds are produced. The fermented solution is then filtered under high pressure, pasteurized and clarified before it is bottled.
The flavor formation process by yeast in the production of soy sauce is lengthy because the yeasts’ metabolisms are slowed down to accommodate the highly saline soy sauce medium. As a result, a large amount of research has been performed in recent decades to attempt to discover ways to enhance or speed it up. This research has consequentially helped shed light on how it is that yeasts like Z. rouxii make the flavors that are so characteristic of soy sauce. There are three main flavor compounds that are produced by Z. rouxii in soy sauce fermentation – Ethanol, higher alcohols (isobutyl, isoamyl, etc.), and 4-hydroxyfuranone derivatives.
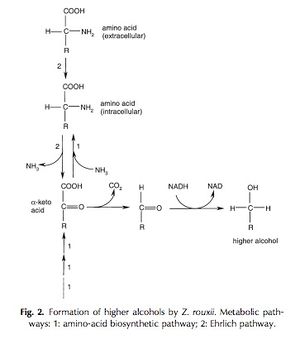
Ethanol production is probably the most straightforward process of the three flavor production pathways that Z. rouxii performs in soy sauce fermentation. The cells use the classic ethanol fermentation pathway to convert sugars in the intermediate brine solution to ethanol. Z. rouxii typically can ferment maltose and fructose in addition to glucose, but the high NaCl concentration of the brine solution limits the yeast to only metabolize glucose. Interestingly enough, ethanol fermentation by Z. rouxii has been observed to only occur when the extracellular environment has dropped to a pH less than, or equal to, ~5.[11] For reasons that are still unclear, at a pH any higher than 5, Z. rouxii cannot maintain the proper H+ gradient that is required for it to continue to be halotolerant.
Unlike the formation of ethanol as a flavor compound in soy sauce fermentation, the production of higher alcohols by Z. rouxii is not that simple. Alcohols like 2-phenylethanol, isoamyl alcohol, methionol, and isobutyl alcohol are all produced by Z. rouxii and provide various flavors to the fermenting soy sauce, adding a certain viscosity to the product. Researchers have determined that the main intermediate compounds involved in the production of these higher alcohols are α-keto acids (Figure 4).[11] The α-keto acids are decarboxylized and then reduced to create the higher alcohols, but the acid intermediates themselves can be created in two different ways (Figure 4). The first process is the amino-acid biosynthetic pathway, and the second is the Ehrlich pathway, which is what researchers suspect is the main pathway used by Z. rouxii.[12] The Ehrlich pathway involves the consumption, and deamination or transamination of exogenous amino acid resources.[12] These extracellular amino acids are extremely prevalent in the brine fermentation mixture. As a result the Ehrlich pathway is much more efficient that the biosynthetic one for the yeast, especially considering the energy consumed to maintain osmotic homeostasis in the brine.
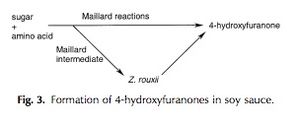
Likely the most important of the main flavor compounds in soy sauce, 4-hydroxyfuranones have been recently observed as biosynthetic products of Z. rouxii. More specifically, the derivatives of 4-hydroxyfuranone – 4-hydroxy-2,5-dimethyl-3(2H)-furanone (HDMF) and 4-hydroxy-2(or 5)-ethyl-5(or 2)-methyl-3(2H)-furanone (HEMF) – were observed by researchers during the Z. rouxii brine fermentation period.[13] These compounds are responsible for the subtle sweetness and “burnt-caramel” flavor of the finished soy sauce. Despite many efforts to uncover the mechanism by which Z. rouxii biosynthesizes these 4-hydroxyfuranones, it remains unclear. The cause of this lack of understanding is twofold. First, the majority of these flavor compounds are actually created in fermented foods like soy sauce or miso when sugars and amino acids in the fermenting compounds spontaneously react. The instances when Z. rouxii is responsible for synthesizing 4-hydroxyfuranones are the minority of the cases. Second, when Z. rouxii does in fact participate in the synthesis of the furanones, it does so using intermediates from the spontaneous – Maillard – reactions that occur in the solution (Figure 5).[11]
Enhancing Soy Sauce with Z. Rouxii Genetics
As discussed above, during the brine fermentation stage of soy sauce production, Z. rouxii synthesizes the various flavor compounds that are critical to the distinct taste associated with soy sauce. However, the unique halo- and osmo-tolerance of Z. rouxii that allow for it to be so productive in the unforgiving environment of the moromi come at a price to soy sauce producers. While Z. rouxii can metabolize sugars in the extremely saline medium, they do so quite inefficiently, which is why the brine fermentation process can take at least 6 months to complete.[11] Additionally, because the yeasts function at a reduced metabolic rate, the flavor compounds they are producing are a much lower concentrations than they could be. [14] When producing soy sauce at a large scale, this inefficiency in flavor production can prove to be somewhat costly. Therefore, researchers are beginning to look into ways to manipulate Z. rouxii in order to enhance the flavor in soy sauce.
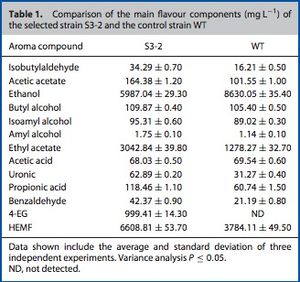
One prominent hypothesis in this topic of research is that enhanced flavor compound production could be achieved by mutating the wild-type (WT) strain of Z. rouxii. For instance, a group of researchers in 2009 sought to utilize genome shuffling of the WT to increase its already very high halo-tolerance, which would in turn allow the yeasts to produce flavor compounds at a much higher rate and concentration, thus improving the taste of the soy sauce.[14] In their study, they induced mutation in the WT with ethyl methanesulfonate (EMS) in order to create a starting population of halo-tolerant variants of Z. rouxii. The researchers then selected the mutants with the strongest growth on yeast extract peptone dextrose plates with NaCl concentrations above 190g/L (the MIC for the WT Z. rouxii).[14] The most successfully mutated strain in terms of increased halo- and osmo-tolerance after the genome shuffling process was Z. rouxii S3-2. Soy sauce was then produced with S3-2 and compared to a control batch using the WT. The scientists determined a 13.33% reduction in the brine fermentation period in the S3-2 batch relative to the WT batch.[14] This partially confirmed the hypothesis that a mutated strain with higher halo-tolerance could ferment more efficiently, but what was even more supportive were the results of the flavor compound break down in the two batches. As Figure 6 shows, the S3-2 strain produced significantly improved concentrations of butyl alcohol, ethyl acetate, propionic acid, benzaldehyde, amyl alcohol, acetic acetate. The S3-2 mutant also increased the concentration of the most important flavor compound, HEMF, by up to 75% as compared to the WT strain.[14] This study, which was the first of its kind, provided significant evidence to support the hypothesis of improved Z. rouxii fermentation via mutation. This kind of research is paramount for the continued enhancement of traditional food production practices that involve microbial life, like the brewing of soy sauce.
Conclusion
Zygosaccharomyces rouxii is a halo- and osmo-tolerant, fructophilic spoilage and fermenting agent. These unique characteristics stem from a variety of physiological and metabolic genetic expressions and adaptations. These adaptations provide advantages to the microbe in terms of where it can survive, even though that may come at the loss of some of our favorite non-perishable food items. These adaptations also allow for Z. rouxii, when in the proper environment, to produce wonderful ingredients like soy sauce that are a cultural staple for a huge portion of the human population. In the characteristics of Z. rouxii we see a story of extremes. It is the microbe that can live in extreme salinity or sugar, and spoil your food, or at the same time produce the subtle and nuanced characteristic flavors we appreciate so much in soy sauce and other fermented foods. If anything Z. rouxii provides us with an underappreciated example of the vast versatility of microbial life, and a fresh perspective on its interaction with human existence in the polar opposite ways in which Z. rouxii affect our food.
Supplemental Figures
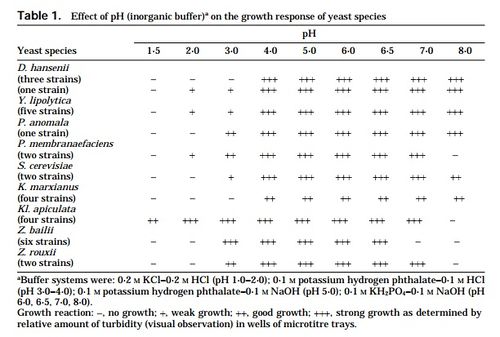
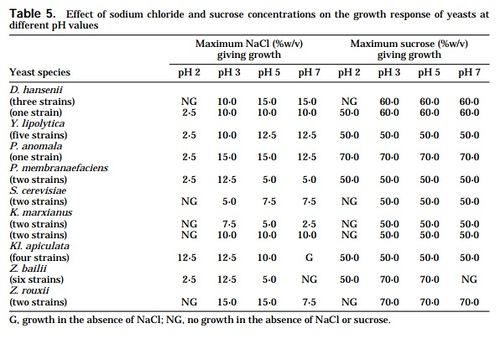
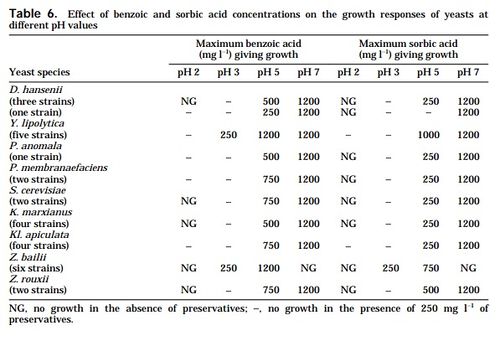
References
- ↑ 1.0 1.1 1.2 1.3 1.4 Dakal TC., et al., 2014. “Adaptive response and tolerance to sugar and salt stress in the food yeast Zygosaccharomyces rouxii.” Int J Food Microbiol.185: 140-157.
- ↑ 2.0 2.1 Leandro, MJ., et al., 2011. “The osmotolerant fructophilic yeast Zygosaccharomyces rouxii employs two plasma-membrane fructose uptake systems belonging to a new family of yeast sugar transporters.” Microbiology 157(2):601-608.
- ↑ 3.0 3.1 3.2 Tokuoka, K., 1993. “Sugar- and Salt-Tolerant Yeasts.” J. Gen. Appl. Microbiol. 74, 101–110.
- ↑ Klis, FM., et al., 2006. “Cell wall construction in S. cerevisiae”. Yeast 23, 185–202.
- ↑ Hamada, T., et al., 1984. “Structure of cell wall and extracellular mannans from Saccharomyces rouxii and their relationship to a high concentration of NaCl in the growth medium.” Appl. Environ. Microbiol. 48, 708–712.
- ↑ 6.0 6.1 Pribylova, L., et al., 2007. “Osmoresistant yeast Zygosaccharomyces rouxii: the two most studied wild-type strains (ATCC 2623 and ATCC 42981) differ in osmotolerance and glycerol metabolism.” Yeast 24, 171-180.
- ↑ 7.0 7.1 Rodicio, R. & Heinsich, JJ., 2010. “Together we are strong—cell wall integrity sensors in yeasts.” Yeast 27, 531-540.
- ↑ Hohmann, S., 2002. Osmotic stress signaling and osmoadaptation in yeasts. Microbiol. Mol. Biol. Rev. 66, 300–372.
- ↑ 9.0 9.1 9.2 9.3 Praphailong, W. & Fleet GH., 1997. “The effect of pH, sodium chloride, sucrose, sorbate and benzoate on the growth of food spoilage yeasts.” Food Microbiology 14, 459-468.
- ↑ 10.0 10.1 10.2 Martorell, P., et al., 2006. “Physiological characterization of spoilage strains of Zygosaccharomyces bailii and Zygosaccharomyces rouxii isolated from high sugar environments.” International Journal of Food Microbiology 114, 234–242.
- ↑ 11.0 11.1 11.2 11.3 11.4 11.5 11.6 11.7 van der Sluis, C., et al., 2001. “Enhancing and accelerating flavour formation by salt-tolerant yeasts in Japanese soy-sauce processes.” Trends in Food Science & Technology 12, 322-327.
- ↑ 12.0 12.1 van der Sluis, C., et al., 2000. “Effect of threonine, cystathionine and the branched-chain amino acids on the metabolism of Zygosaccharomyces rouxii.” Enzyme Microb. Tech. 26, 293–301.
- ↑ Hauck, T., et al., 2003. “Formation of 4-Hydroxy-2,5-Dimethyl-3(2H)-Furanone by Zygosaccharomyces rouxii: Identification of an Intermediate.” Applied and Environmental Microbiology 69, 3911-3918.
- ↑ 14.0 14.1 14.2 14.3 14.4 14.5 Cao, X., Hou, L., Lu, M., Wang, C. and Zeng, B. (2010), "Genome shuffling of Zygosaccharomyces rouxii to accelerate and enhance the flavour formation of soy sauce." J. Sci. Food Agric., 90: 281–285.
Authored for BIOL 238 Microbiology, taught by Joan Slonczewski, 2017, Kenyon College.