Spoiler Alert: Zygosaccharomyces Rouxii and It's Role in Food Spoilage and Fermentation
Introduction
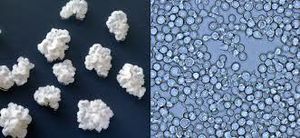
By Daniel Maffezzoli
Zygosaccharomyces rouxii is an interesting species of yeast that is infamous within the food industry. Its capacity to thrive in both highly saline and sugar-dense environments is what makes Z. rouxii a predominant food spoiling agent. The metabolism and ruggedness of the microbe also allow it to resist typical preservative methods in food production. In addition to being a spoiling agent, Z. rouxii is actually used to create various staple ingredients and traditions in East Asian cuisine. More specifically, Z. rouxii is the principal microbe used in the fermentation of soybeans during the brewing process of soy sauce, and in the production of miso. This page aims to provide detailed information on the unique metabolism and homeostatic tendencies of this microbe, how the microbe goes about spoiling foods, and the productive qualities that arise from the spoilage-inducing features – particularly in the context of brewing soy sauce.
Habitat, Physiology, and Metabolism
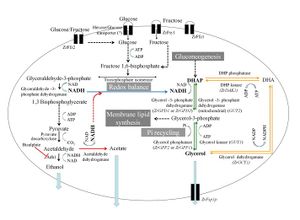
The relationship between habitat and metabolism is typically the most telling aspect of any microbe. The habitat of a given microbe can give large clues about how its metabolism functions, and vice versa. What makes the habitat-metabolism relationship in Z. rouxii so unique is not only that its most ideal growth conditions are extreme by conventional standards, but also that it makes a home in food products that are generally considered to be “non-perishables.” Z. rouxii is the most prominently found microbe in spoiled syrups, honey, fruit juice concentrates, jams, jellies, sauces, sodas, and various shelf-stable condiments like ketchup.[2]
The capacity for Z. rouxii to grow in these microbe-unfriendly environments is a direct result of the osmotolerant and halotolerant properties of its metabolic pathways. It is important to differentiate these characteristics due to the fact that each distinct solute results in a specific corresponding stress response by the microorganism. Z. rouxii is one of the few yeast species that seems to have minimal stress responses to the two conventional environmental stressors of high glucose and high salinity. Researchers have observed Z. rouxii growth in conditions of up to 65%(w/v) D-glucose and 25%(w/v; 4.5M) NaCl which amounts to water activity (aw) values of 0.88 and 0.85, respectively.[3] [4] In addition to its osmotic adaptability, Z. rouxii is fructophilic, which allows for more diverse sources of energy. The ability to tolerate such high levels of extracellular solutes and the stresses that accompany them can be traced to the three main regulatory physiological and metabolic pathways in yeasts – the alteration of the cell wall and plasma membrane, changes to the transport systems of the cell, and equilibration of metabolic osmolytes.[1]
The structure of the cell wall and plasma membrane of a yeast cell is integral to its tolerance of high osmotic pressure and salinity. Yeasts are capable of reshaping their morphological composition in order to counter the osmotic pressure of their extracellular environments. The sturdy cell wall of a yeast microbe is comprised of three categories of molecules: mannan, glucan and chitin.[5] Initial research determined that the level of mannans in the Z. rouxii cell wall decreased in the presence of high extracellular NaCl content.[6] The results of the studies like these presented a correlation between cell wall thickness and halotolerance, but more recent strain-specific studies determined that certain strains of Z. rouxii with more malleable cell walls were actually more halotolerant than strains with rigid walls.[7] These results clearly suggest a relationship between cell wall mannan and glucan composition and cell wall integrity, although there is further research to be done to reinforce this morphological stress tolerance mechanism. However, the regulatory pathway that induces changes in the cell wall in Z. rouxii has been fairly well researched using S. cerevisiae as a model.[8] In S. cerevisiae the primary pathway for regulating cell wall rigidity is the cell wall integrity signaling pathway (CWISP). Since there is evidence to support the fact that this pathway is rooted in the genetic expression of the yeasts, and Z. rouxii is the Zygosaccharomycete most phylogenically similar to S. cerevisiae, it has been suggested in genetic research that this pathway is likely the one used by Z. rouxii.[9] The CWISP is triggered when osmotic stress is induced. When sensor proteins on the cell wall are activated, signals are sent to the enzyme Rho1 GTPase.[1] This enzyme then triggers the kinesis of multiple effectors, which, depending on the character of the initial stimulus, either up- or down-regulate the production and transport of the structural polymers discussed earlier like mannans and glucans to fortify or reduce the cell wall’s integrity. The CWISP has also been observed as a response to mechanical stress stimuli and simple cell wall maintenance.[1]
The second physiological and metabolic pathway that plays a role in the osmotolerance of Z. rouxii is its use and modulation of sugar transporters. Unlike S. cerevisiae, which takes in hexoses by way of facilitated diffusion when in a glucose-depleted hyperosmotic environment, Z. rouxii is a fructophilic microbe and actually prefers a fructose-rich extracellular environment. As pictured in Figure 1, which depicts the variety of metabolic pathways of fructose and glucose that Z. rouxii possesses, the yeast employs two fructose and glucose uptake facilitators when the external levels of those sugars are high. Additionally, researchers in Portugal recently determined that Z. rouxii makes use of a high-affinity, but low-capacity fructose transport protein that is up-regulated when the yeast is in growth medium with low sugar concentration.[10]
The regulation of sugar transporters leads to the final regulatory pathway involved in the high levels of tolerance of Z. rouxii when grown in unfavorable environments, which is the production and sequestration of metabolic osmolytes. The balance of osmolytes is integral to maintaining various homeostatic thresholds like water balance and pH. The principal osmolyte that is used to maintain homeostasis in hyperosmotic environments is glycerol. In general, there are two main metabolic pathways of glycerol that yeasts use (both pictured in Figure 1): the Gcy-Dak pathway and the Gpd-Gpp pathway. The Gpd-Gpp pathway is the primary metabolic pathway utilized by Z. rouxii. In S. cerevisiae, glycerol retention is integral to the maintenance of intracellular water content when responding to osmotic stress. As a result, the cells will biosynthesize large quantities of the molecule, which is inefficient because most of it is lost to the extracellular medium because of the concentration gradient.[11] In Z. rouxii, however, we see something different, which is that the cells will biosynthesize much smaller amounts of glycerol and instead decrease the permeability and pliability of their plasma membranes via manipulated surface phospholipid compositions.[12] This strategy ends up being much more effective as a response to hyperosmotic stress than that of S. cerevisiae and can be connected to the ability for Z. rouxii to survive in hyperosmotic or saline environments.
In addition to hyperosmosis, there are other environmental stress factors that Z. rouxii withstands substantially. The supplemental tables provided at the bottom of the page demonstrate the range of pH, NaCl, sorbate and benzoate levels in which Z. rouxii showed significant positive growth.[13]
Food Spoilage
Include some current research, with at least one figure showing data.
Soy Sauce Prodcution
Include some current research, with at least one figure showing data.
Conclusion
Supplemental Figures
References
- ↑ 1.0 1.1 1.2 1.3 Cite error: Invalid
<ref>
tag; no text was provided for refs namedDakal
- ↑ Leandro, MJ., et al., 2011. “The osmotolerant fructophilic yeast Zygosaccharomyces rouxii employs two plasma-membrane fructose uptake systems belonging to a new family of yeast sugar transporters.” Microbiology 157(2):601-608.
- ↑ Tokuoka, K., 1993. “Sugar- and Salt-Tolerant Yeasts.” J. Gen. Appl. Microbiol. 74, 101–110.
- ↑ Dakal TC., et al., 2014. “Adaptive response and tolerance to sugar and salt stress in the food yeast Zygosaccharomyces rouxii.” Int J Food Microbiol.185: 140-157.
- ↑ Klis, FM., et al., 2006. “Cell wall construction in S. cerevisiae”. Yeast 23, 185–202.
- ↑ Hamada, T., et al., 1984. “Structure of cell wall and extracellular mannans from Saccharomyces rouxii and their relationship to a high concentration of NaCl in the growth medium.” Appl. Environ. Microbiol. 48, 708–712.
- ↑ Pribylova, L., et al., 2007. “Osmoresistant yeast Zygosaccharomyces rouxii: the two most studied wild-type strains (ATCC 2623 and ATCC 42981) differ in osmotolerance and glycerol metabolism.” Yeast 24, 171-180.
- ↑ Rodicio, R. & Heinsich, JJ., 2010. “Together we are strong—cell wall integrity sensors in yeasts.” Yeast 27, 531-540.
- ↑ Cite error: Invalid
<ref>
tag; no text was provided for refs namedRodicio
- ↑ Cite error: Invalid
<ref>
tag; no text was provided for refs namedLeandro
- ↑ Hohmann, S., 2002. Osmotic stress signaling and osmoadaptation in yeasts. Microbiol. Mol. Biol. Rev. 66, 300–372.
- ↑ Cite error: Invalid
<ref>
tag; no text was provided for refs namedPrib
- ↑ Praphailong, W. & Fleet GH., 1997. “The effect of pH, sodium chloride, sucrose, sorbate and benzoate on the growth of food spoilage yeasts.” Food Microbiology 14, 459-468.
Authored for BIOL 238 Microbiology, taught by Joan Slonczewski, 2017, Kenyon College.