Carbon cycle: Difference between revisions
Line 64: | Line 64: | ||
[[Image:Greehouse_Effect.jpg|frame|center|The Greenhouse Effect. From [http://sitemaker.umich.edu/section9group2/introduction]]] | [[Image:Greehouse_Effect.jpg|frame|center|The Greenhouse Effect. From [http://sitemaker.umich.edu/section9group2/introduction]]] | ||
====Water Vapour==== | ====Water Vapour==== | ||
"Water vapor is a naturally occurring greenhouse gas and accounts for the largest percentage of the greenhouse effect, between 36% and 66%. The water vapor content of the atmosphere is expected to increase in response to an increase in temperatures. The increased water vapor in turn leads to an increase in the greenhouse effect and thus a further increase in temperature; the increase in temperature leads to still further increase in atmospheric water vapor; and the feedback cycle continues until equilibrium is reached. Thus water vapor acts as a positive feedback to the driving force provided by human-released greenhouse gases such as CO<sub>2</sub>" [5]. | "Water vapor is a naturally occurring greenhouse gas and accounts for the largest percentage of the greenhouse effect, between 36% and 66%. The water vapor content of the atmosphere is expected to increase in response to an increase in temperatures. The increased water vapor in turn leads to an increase in the greenhouse effect and thus a further increase in temperature; the increase in temperature leads to still further increase in atmospheric water vapor; and the feedback cycle continues until equilibrium is reached. Thus water vapor acts as a positive feedback to the driving force provided by human-released greenhouse gases such as CO<sub>2</sub>" [5][8]. | ||
====Nitrous Oxide==== | ====Nitrous Oxide==== |
Revision as of 08:45, 24 March 2008
Introduction
The Carbon Cycle affects all life on Earth. Elemental carbon is neither created or destroyed, so the recycling of carbon-containing compounds in the environment is essential to the continuation of life. Recently there has been increased interest in the Carbon Cycle because of our awareness of how atmospheric levels of carbon dioxide (CO2) affect global climate change. Microbes play important roles in all aspects of the Carbon Cycle and are a very important consideration when analyzing climate change. Many produce CO2 through respiration,and some fix atmospheric carbon, sequestering it in the soil. Some produce methane and nitrous oxide, which are potent greenhouse gases, while others degrade toxic and environmentally harmful compounds.
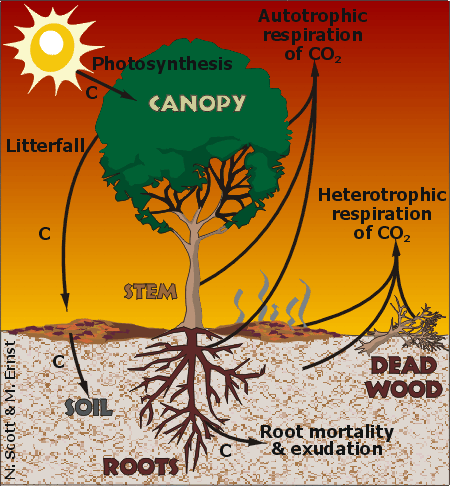
The Carbon Cycle
Carbon Fixation
An important step of the carbon cycle is the fixation of atmospheric CO2 and its subsequent assimilation into organic molecules. Organisms that can convert CO2 into organic molecules are called autotrophs, and include plants, algae, some bacteria, and some archaea. Some bacteria specifically involved in carbon fixation are Cyanobacteria (such as Chroococcus, Nostoc, and Spirulina) and some species of Aquifex,such as Aquifex aeolicus. Cyanobacteria in the soil are also important Nitrogen fixers in the Nitrogen cycle including GHG.
Decomposition of Organic Matter and the Microbes Involved
The major function of microbes in the Carbon Cycle is as decomposers-- degraders of complex organic molecules that would otherwise permanently sequester carbon, keeping it from being useful to organisms. Many of these decomposers also release CO2, contributing to the rising concentration in the atmosphere. Each organic compound is utilized in a slightly differently manner, and each yields different products when degraded.
Sugars and Proteins
Plant debris supplies most of the simple sugars and proteins in the soil. All heterotrophic microbes can easily utilize these compounds for both energy metabolism and as carbon sources. Plants link glucose molecules (and other sugar monomers) into long chains to produce polymers such as starch and cellulose, which require more specialized organisms to degrade.
For example: Starch is made of amylose (alpha 1,4 bonded glucose) and amylopectin (alpha 1,6 bonded glucose), and is relatively easy for most organisms to degrade. Cellulose, however, is a polysaccharide of glucose connected with beta 1,4 bonds, and is more difficult to degrade. No animal can degrade cellulose, so bacteria can frequently be found in mutualistic relationships with detritivores: the bacteria degrades the cellulose enough that the animal is able to digest it.
Cellulose
Cellulose is a structural polysaccharide that contains glucose. In order to access this glucose for catabolism, the cellulose must be cleaved by extracellular enzymes. These pieces are then transported into the cell for energy generation (catabolism) or production of biomass (anabolism). Fungi such as Penicillium and Aspergillus and bacteria such as Streptomyces and Pseudomonas are important participants in the extracellular cleavage of cellulose [2].
Hemicellulose
Hemicellulose is the next most common carbohydrate source in plants. The decomposition of hemicellulose is similar to that of cellulose in that the initial depolymerization step takes place outside of the cell, and the sugars produced are then transported into the cell for catabolism or anabolism. Even though hemicellulose decomposition is much quicker than cellulose decomposition, cells will utilize simple sugars as substrates before hemicellulose [2].
Chitin
Chitin is a special compound which can be found in the integument of arthropods and cell wall of fungi. The polymer is not easily degraded,and requires a variety of enzymes to do so. The dominant chitin degraders are the actinomycetes Streptomyces and Nocardia, and (less importantly) fungi such as Trichoderma and Verticillium.
Lignin
Lignin is the main component of wood in trees. Lignin has a varied, unique, and complicated chemical structure which contains many aromatics. These aromatics can be released from the lignin sturucture by fungal enzymes such as peroxidases and oxidases. The enzymes utilize H2O2 and OH radicals to break the bonds in the lignin. Common types of fungi which degrade lignin are white rot (Phanerochaete chrysosporium), brown rot, and soft rot. Once the aromatics are released from the original lignin structure they are incorporated into the metabolic pathway as pyruvate, acetyl CoA, and into the TCA cycle.
The modification and breakdown of lignin by fungal and bacterial enzymes help contribute to the formation of humus.
Aromatics, Aliphatics, and Lipids
Aromatics are compounds released when bonds in lignin are broken by fungal enzymes. The aromatics enter or are incorporated as pyruvate, acetyl CoA, and processed in the TCA cycle. Aliphatics are non-aromatic organic compounds and are incorporated into the TCA cycle after being converted into acetyl CoA.
Soil Organic Matter
The soil organic matter (SOM) is a complex combination of living organisms, fresh organic residues, actively decomposing material, and stabilized organic matter (otherwise known as humus). Generally, samples containing 50% or more of carbon are refered as soil organic carbon (SOC).
Where Does SOM come from
SOM comes either from plant and crop residues, such as stems, leaves, or shoots, or indirectly from sewage or manure.
The "living" part of SOM includes bacteria, fungi, and insects; the "nonliving" part of SOM comes from recently dead plant or animal matter, and excreta from soil organisms.
Humus
Humus is a combination of many compounds, and is critical in defining many properties of a soil. It increases water and nutrient retention, encourages aggregate formation, and inhibits impaction and caking. Humus is composed mainly of humin, humic acid, and fulvic acid. Two major methods of humus formation are by minor modifications of lignin and by the degradation and reassembly of plant residues (lignin, cellulose, hemicellulose) by fungal extracellular enzymes. Humus is a very stable, long-lived pool of organic matter in the soil (with a turnover rate of 100-500 years), which makes it an effective way to sequester excess carbon.
Benefits of Humus in the Soil
1. Increases the ability of the soil to store water.
2. Absorbs and deactivates many chemicals such as herbicides.
3. Increases the resistance from attack by microorganisms.
4. Makes the soil richer in nutrients.
Atmospheric Changes Relating to the Carbon Cycle
Global Warming
Global warming is a result of a greenhouse effect caused by the accumulation of greenhouse gases in the atmosphere. The earth is heated by solar radiation that penetrates the atmosphere and is absorbed by the earth. Some of the enegy is reflected back into the atmosphere. Greenhouse gases prevent the escape of this solar energy, causing increased heating of the earth, a phenomenon known as global warming. The major greenhouse gases are water vapour, nitrous oxide (N2O), carbon dioxide (CO2), methane (CH4), and ozone (O3). Soil microorganisms play a role in the generation of each of these gases [3].
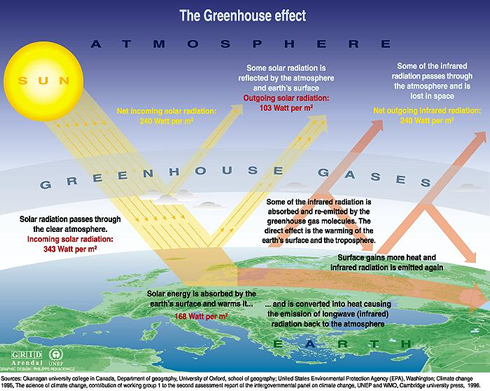
Water Vapour
"Water vapor is a naturally occurring greenhouse gas and accounts for the largest percentage of the greenhouse effect, between 36% and 66%. The water vapor content of the atmosphere is expected to increase in response to an increase in temperatures. The increased water vapor in turn leads to an increase in the greenhouse effect and thus a further increase in temperature; the increase in temperature leads to still further increase in atmospheric water vapor; and the feedback cycle continues until equilibrium is reached. Thus water vapor acts as a positive feedback to the driving force provided by human-released greenhouse gases such as CO2" [5][8].
Nitrous Oxide
Microorganisms produce N2O by performing aerobic nitrification and anaerobic denitrification. Nitrification is the conversion of ammonia to nitrate. Nitrobacter hamburgensis and Nitrosococcus oceani are examples of nitrifying bacteria. Denitrification is the reduction of nitrate to nitrogen gas, with N2O as a side product. Pseudomonas and Flavobacterium are examples of denitrifying bacteria [2]. For more information on nitrogen transformation, see the Nitrogen cycle including GHG.
Carbon Dioxide
Microorganisms produce CO2 through the decomposition of organic matter. The balance between microbial repiration of CO2 and plant fixation of CO2 is important when considering microbial contribution to the greenhouse effect [2].
Methane
Methane is the end product of anaerobic respiration. This process is called methanogenesis. Microorganisms that produce methane through respiration are called Methanogens. There are two processes by which methanogens produce methane: CO2 reduction and acetate fermentation [2].
Methanotrophy is the process by which microorganisms, such as Methylobacterium, utilize methane as a carbon source. Carbon dioxide is a product of the reaction. These microorganisms are called methanotrophs [2].
Acid Rain
Nitrous oxides not only contribute to the greenhouse effect, but also to acid rain. Acid rain is corrosive, and increases the acidity of surface water and soils. Basic soils, including soils containing substantial amounts of calcium carbonate, can neutralize the acid rain. These soils are common in the Midwest, Great Plains, and many Western states [1].
Surface water becomes acidified when the buffering capacity of the water and soil is overwhelmed by the acid rain. The acid rain liberates aluminum from the soil. The aluminum then enters the water, posing a serious threat to aquatic organisms. Acidification of soils, and the associated release of aluminum, also poses a threat to soil microorganisms. Low pH can also release toxic metals such as lead, mercury, zinc, copper, cadmium, chromium, manganese, and vanadium from the soil. These mobilized ions inhibit the growth of soil bacteria and fungi [1].
The effects of acid rain also accelerate calcium carbonate (CaCO3) degradation. CaCO3 reacts with the acid to form CaO and CO2. The CO2 is released from the soil and combines with water vapor in the atmosphere to form more acid rain. This process, which continues until CaCO3 is completely consumed, is an example of a positive environmental feedback loop.
Current Research
Professor Joshua Schimel is studying the effect of global warming on the soil organic matter in the Arctic. The increasing temperature could result in increased evolution of CO2 to the atmosphere. On the other hand, plant growth could be fostered by the increased presence of nutrients from soil organic matter, making the Arctic a sink for CO2. The nature of the organic matter present in the Arctic soils will determine the type of environmental feedback. http://www.lifesci.ucsb.edu/eemb/faculty/schimel/research/research.html
The Urban Water Research Center at UC Irvine is conducting research on the effect of stengthened anaerobic degradation of manure on methane generation. http://www.uwrc.uci.edu/research-urban-water/UCI-UWRC-Research-Areas.php
Recently the EPA released a report analyzing the existing data on the connections between UV radiation, global climate change, and biogeochemical cycling. The report found that increased UV radiation affected the cycling of nutrients such as carbon and nitrogen. UV radiation impacts root exudation and the properties of dead plant material that become incorporated into the soil. This impacts microorganisms, and in turn, biogeochemical cycling. http://www.ncbi.nlm.nih.gov/pubmed/17344963?ordinalpos=1&itool=EntrezSystem2.PEntrez.Pubmed.Pubmed_ResultsPanel.Pubmed_DiscoveryPanel.Pubmed_Discovery_RA
References
1."Acid Rain - Soil Interactions" Available from: http://www.elmhurst.edu/~chm/vchembook/196soil.html
2.Sylvia, D.M., Fuhrmann, J.J., Hartel, P.G.,& Zuberer, D.A. (2005).Principles and Applications of Soil Microbiology, Second Edition. Prentice Hall, Upper Saddle River, NJ, 346,359,497-501 and 285-287 pp.
3.http://www.blackwell-synergy.com/doi/abs/10.1046/j.1365-2486.1997.00100.x KEITH SMITH (1997) "The potential for feedback effects induced by global warming on emissions of nitrous oxide by soils" Global Change Biology 3 (4) , 327–338 doi:10.1046/j.1365-2486.1997.00100.x
4.http://www.lifestyleblock.co.nz/articles/organics/02_organic_soil_matter.htm
5.realclimate.org http://web.archive.org/web/20070624015308/http://www.realclimate.org/index.php?p=142 "Water vapour: feedback or forcing?"
6.http://www.gfdl.noaa.gov/reference/bibliography/2006/ih0601.pdf Issac M. Held, Brian J. Soden. (2006) "Robust Responses of the Hydrological Cycle to Global Warming " Journal of Climate volume19,issue = 21,pages5686-5699
7.Erickson DJ 3rd, Paul ND, Sulzberger B, Zepp RG. "Interactive effects of solar UV radiation and climate change on biogeochemical cycling," Photochem Photobiol Sci. 2007 Mar;6(3):286-300. Epub 2007 Feb 6.PMID: 17344963 [PubMed - indexed for MEDLINE] http://www.ncbi.nlm.nih.gov/pubmed/17344963?ordinalpos=1&itool=EntrezSystem2.PEntrez.Pubmed.Pubmed_ResultsPanel.Pubmed_DiscoveryPanel.Pubmed_Discovery_RA
Edited by student of Kate Scow